13 Biopolymers
joh28
Learning Objectives
Upon completion of this chapter, students will be able to:
- Define biopolymers and explain their characteristics compared to synthetic polymers.
- Identify fabrication methods for biopolymers, such as fermentation, isolation, and chemical synthesis, and describe their processes.
- Compare and contrast the structure and material properties of different biopolymers.
- Propose applications of biopolymers based on their structure and properties.
1. Introduction
The development of petrochemical materials has brought convenience to modern society but it has also led to significant environmental concerns. From pollution caused by the materials themselves to the impacts of production and disposal, traditional plastics have raised global concerns. With the increasing annual plastic production and only a fraction of the waste effectively recycled, the need for sustainable alternatives has become crucial. These concerns have renewed interest in using natural, bioderived materials and developing biopolymers as an alternative to traditional petrochemical plastics.
Biopolymers are materials derived from biological compounds, typically gained from renewable resources. Some biopolymers are biodegradable, which makes them particularly suitable for addressing environmental challenges. These materials can potentially reduce dependence on petrochemical resources while mitigating the environmental impacts of traditional plastics. Furthermore, biopolymers are often compatible with existing waste management systems, making them suitable for various industries. A deeper understanding of the relationship between the structure and properties of biopolymers is critical to the further development of bioplastic materials and their applications.
Fabrication methods are a key aspect of understanding biopolymers. Various techniques have been developed to successfully get biopolymers from natural organic compounds. These include fermentation processes (used for PLA or PHA), direct extraction or isolation (used for cellulose or chitin), and chemical synthesis for modified biopolymers. For biopolymers, these processes are important as the fabrication process influences the properties or performance of resulting materials. Thus, identifying the optimal fabrication method for each biopolymer ensures that these materials perform comparably to traditional plastics while maintaining their sustainability.
The application of biopolymers has great potential across diverse industries, including textiles, packing, agriculture, and biomedical fields. For example, PLA’s biodegradability makes it suitable for single-use items like compostable bags or cups and food packaging, while chitosan’s antimicrobial properties make it ideal for wound dressings. By considering both polymer structure and material performance, bioplastic materials can be designed to meet the demands of various applications.
In this chapter, the concept of biopolymers, including their fabrication methods, structure, properties, and applications will be explored. This chapter aims to provide a comprehensive understanding of how biopolymers are produced and how their molecular structures enable their use in sustainable applications. By focusing on recent advancements, this chapter highlights the importance of biopolymers in addressing global challenges and offering a path toward a more sustainable future.
2. Biopolymers
Biopolymer refers to organic materials derived from natural or biological sources. Most biopolymers are recognized for their biocompatibility and environmental sustainability. The term “biopolymer” is often used interchangeably with “bioplastics”, but it is essential to distinguish between the two. Bioplastics may refer to either biodegradable plastics (including biodegradable plastics derived from petrochemical sources) or bio-based plastics (produced from biological sources) [1]. In this chapter, biopolymers specifically refer to macromolecules derived, extracted, or synthesized from biological sources, emphasizing their sustainability and biological origins.
Usually, biopolymers are classified as either natural polymers, which are found naturally in their environment (e.g., cellulose from plants or chitin from crustaceans), or bio-based polymers, which are synthetically produced using natural resources (e.g., polylactic acid (PLA) from corn or sugarcane starch). Common examples include monomers like amino acids, sugars, or nucleotides, forming biopolymers such as chitin, starch, cellulose, peptides, proteins, DNA, and RNA [2]. Based on their origin, biopolymers can be categorized into natural, synthetic, and microbial types [2].
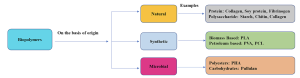
Biopolymers differ from synthetic petroleum-based polymers and traditional plastics in several significant ways. Their unique properties make them more biocompatible, sustainable, and often biodegradable. One of the most distinctive characteristics is their origin, as biopolymers are from natural biological sources. Plants, agricultural crops, microbial sources, animals, and agricultural waste are examples of natural sources of biopolymers. Common sources of biopolymers include plants or crops (corn, potatoes, cotton), microbial sources (algae, fungus, yeast), animals (crustaceans, corals), and agricultural waste (paper or wood waste). Additionally, most bio-derived polymers are biodegradable, meaning that they can be broken down into smaller molecules through biochemical actions, usually from microorganisms.
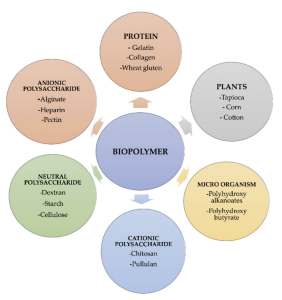
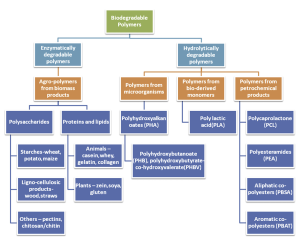
The history of biopolymers dates back to ancient times when materials like natural rubber and cellulose were used for basic needs. Modern research into biopolymers and their application as a sustainable alternative to petroleum-based plastics began in the mid-20th century due the increasing environmental concerns. For example, chitin, a common biopolymer, was first discovered in 1799, and its exploration gained focus during the mid-20th century [4]. Significant advancements during this period include the microbial production of polylactic acid (PLA), which became a prominent example of biodegradable bioplastics. In recent years, research has expanded to include engineered biopolymers or biocomposite, and established biopolymers as important areas of innovation in the field of sustainable materials [5].
Biopolymers can be broadly classified into three categories based on their composition: polysaccharides, proteins, and polyesters. The first group, polysaccharides, includes cellulose, chitin, and starch. Cellulose is one of the most abundant biopolymers, which is most widely used in textiles and paper due to its strength and rigidity. Another polysaccharide, chitin is derived from crustacean shells (e.g., shrimp or crabs), insect exoskeletons, or fungi and is used in wound dressings and water purification. The second group, proteins, includes collagen, soy protein, and fibrinogen. Collagen is used in cosmetics, pharmaceuticals, and regenerative medicine due to its biocompatibility and elasticity. The third group, polyesters, includes polylactic acid (PLA) and polyhydroxyalkanoates (PHA). PLA is produced through the fermentation of sugars and is used in food packaging and 3D printing. Also, PHAs are biodegradable polyesters synthesized from bacteria and suitable for medical implants and agricultural films. These examples illustrate the diversity of biopolymers and their potential to replace traditional plastics across various fields.
3. Fabrication Methods
The fabrication of biopolymers involves diverse processes developed specifically for biological sources and desired applications. These methods include fermentation, isolation, and chemical synthesis. Each of these methods contributes to the advancement of sustainable biopolymer production.
3.1. Isolation
Biopolymers can be directly isolated from natural sources such as plants, animals, or microbial systems. Isolation or extraction is one of the most common methods used for biopolymer preparation. This approach involves separating the macromolecules from biological materials.
Cellulose
Cellulose is one of the most abundant biopolymers and is primarily extracted from plant cell walls. Cellulose polymers can be extracted from sources such as plants (cotton, wood, algae, flax, hemp, bamboo, etc.), agricultural residues (seaweed, rice husk, sugarcane bagasse) [3], or even from tunicates. The isolation of cellulose involves processes such as pulping and bleaching to remove non-cellulosic components like lignin and hemicellulose to obtain purified cellulose. The extraction process can be done through mechanical or chemical methods. The mechanical method uses various equipment and depends on the type of equipment used. For example, high-intensity ultrasonication, high-pressure homogenizers, grinders, blenders, or extruders have been used to extract cellulose [6]. The chemical methods include acid hydrolysis or semi-chemical oxidation processes combined with mechanical treatments [6].
Chitin and Chitosan
Chitin is a structural polysaccharide found in the exoskeletons of crustaceans, insects, and fungi. It is isolated through demineralization (removal of calcium carbonate) and deproteinization (removal of proteins) steps. To extract chitin polymers from natural sources, both mechanical (grinding, ultrasonication, high-pressure homogenizer, blender) and chemical treatments are used [6]. The extracted chitin can be chemically treated to produce chitosan, a partially deacetylated chemical derivative of chitin.
This article on the formation of chitin nanopaers from the mushroom extract demonstrates a sustainable chitin extraction process from the common mushrooms [14]. The schematic figure illustrating the chitin fiber extraction process provides a clear and easily understandable overview. The process starts with mechanical blending to increase the surface area, followed by chemical treatments for alkaline deproteinization. This method is more sustainable compared to conventional extraction processes using crustacean sources. It highlights the potential of mushrooms as a viable source of chitin and the chitin nanopapers show the promising applications of mushroom-derived chitin.
Collagen
Collagen is a structural protein extracted from animal tissues such as skin, tendons, and bones [6]. The extraction of collagen involves chemical (basic, neutral salt, or acid) or enzymatic methods. These methods are used to break down the cross-linked structure in the collagen fibers. The typical collagen isolation process follows three steps: pretreatment (raw materials are pretreated with acid or alkali), collagen extraction (extracted with acetic acid and pepsin), and post-treatment (purified using techniques like dialysis or centrifugation) [6].
3.2. Fermentation
Fermentation is a biological process that uses microorganisms such as bacteria, fungi, or yeast to convert organic sources into biopolymer precursors or directly into biopolymers. This method is particularly known for producing polylactic acid (PLA). The process begins with the fermentation of raw materials like corn starch or sugarcane to produce lactic acid. Lactic acid is the starting material for PLA and can be produced either by fermentation or chemical synthesis routes [7]. Microorganisms such as bacteria, fungi, yeasts, cyanobacteria, and algae are used to produce lactic acid by anaerobically metabolizing the sugars in the feedstocks. Then, lactic acid is polymerized to produce PLA. There are several possible ways to produce PLA from lactic acid, such as direct condensation polymerization or polymerization through lactide formation [8].
3.3. Chemical Synthesis
Chemical synthesis involves the production of biopolymers from bio-based monomers using polymerization techniques. This method offers more control over the molecular structure or molecular weight of the resulting biopolymers. However, most chemical synthesis of biodegradable plastics still uses crude oil and produces aliphatic and aromatic polyesters, polyvinyl alcohol, and modified polyolefins [8]. One example of the chemical synthesis of biopolymers based on natural resources is the chemical synthesis of polylactic acid (PLA) through the polymerization of lactic acid or lactide. Polymerization of PLA through ring-opening polymerization of lactide is widely used to produce high molecular weight PLA [8].
4. Structure and Properties
Biopolymers show a wide range of structures and properties that make them suitable for various applications. The unique structural features of biopolymers, from their chemical composition to molecular structure, include their mechanical and chemical properties. This section provides an overview of the chemical structures and properties of common biopolymers, including cellulose, chitin, chitosan, collagen, and PLA.
Cellulose
Cellulose is a linear polysaccharide composed of repeating units of glucose linked by β-1,4- glycosidic bonds. The abundant hydroxyl (-OH) groups on the glucose units of cellulose enable increased intermolecular hydrogen bonding, which enhances the rigidity and strength of cellulose [9]. The resulting cellulose fibers show high tensile strength, making cellulose a key component in materials such as paper, textiles, and composites. Additionally, the hydroxyl groups allow for chemical modification and production of many derivatives, such as cellulose acetate, which has enhanced solubility and thermal properties.
Chitin and Chitosan
Chitin is the second most abundant natural polysaccharide, following cellulose, and is a linear polymer composed of repeating β(1,4)-N-acetylglucosamine units [10]. The structure of chitin is similar to cellulose but with one hydroxyl group on each glucose unit replaced by an acetyl amine group. This modification makes chitin a strong polymer with increased bonding between the polymer chains. Chitosan is derived from chitin through partial deacetylation of its acetyl amine groups and is composed of d-glucosamine and N-acetyl-d-glucosamine [10]. The free amino groups in chitin give it cationic properties that allow for easy derivatization with improved function. Both chitin and chitosan are rigid polymers with excellent biocompatibility and biodegradability.
Collagen
Collagen is the most abundant protein in mammals, composed of polypeptide chains in a triple-helical structure. As of now, 28 types of collagen have been identified, and the most common type of collagen is Type I found in bones and tendons [6]. Type I collagen is a heterotrimer with three α-polypeptide chains. This unique structure is stabilized by hydrogen bonds and covalent crosslinks, giving collagen excellent strength and flexibility. Collagen is highly elastic, providing strength to tendons and ligaments. As a naturally occurring protein, collagen is also biocompatible and widely used in biomedical applications.
PLA
PLA (polylactic acid) is an aliphatic thermoplastic polyester synthesized from lactic acid by direct condensation or ring-opening polymerization of lactide. It consists of repeating units of lactic acid, and the stereochemistry of lactic acid (L- and D- lactic acid) affects the crystallinity and mechanical properties of PLA. Depending on the type of lactic acid used, several forms of PLA exist, such as poly (l-lactide) (PLLA) and poly (dl-lactide) (PDLLA) [11]. PLA is biodegradable under industrial compositing conditions, typically with enough heat and water. Also, PLA is a widely used bioplastic due to its excellent mechanical and thermal properties.
Biopolymer | Sources | Structure | Ref. |
Cellulose | Plant (cotton, wood, bamboo, flax, hemp, etc.), agricultural residue (Seaweed, rice husk, sugarcane bagasse) | ![]() |
[3] |
Chitin | Crustaceans (crab, shrimp), shells insects, fungi, mushroom | ![]() |
[13] |
Chitosan | Fungi, mollusks, algae, crustaceans, and insects
|
![]() |
[3], [13] |
Collagen | Bones, tendons, corneas, and ligaments | ![]() |
[6] |
PLA | Lactic acid from corn, sugarcane, or other sugar-rich crops | ![]() |
[11] |
5. Applications
Biopolymers have diverse applications due to their unique properties, biocompatibility, biodegradability, and sustainability. They are used in fields ranging from packaging to biomedical engineering, as a environmentally friendly alternative to traditional plastic polymers. The diverse applications of cellulose, chitin, chitosan, collagen, and PLA demonstrate their potential as an alternative to conventional polymers in many industries.
Biopolymer | Applications | Ref. |
Cellulose | Adsorption and separation materials, filtration membranes, biomedicine, optical films | [2], [6] |
Chitin | Water treatment, agriculture, tissue engineering, drug delivery, wound dressings, antimicrobial agents | [6] |
Chitosan | Cosmetics, biomedicine, wound dressing, water treatment, drug delivery, food technology | [12] |
Collagen | Biomedical applications, cosmetics, food packaging | [6] |
PLA | Antimicrobial and acute wound healing, food packaging, agriculture, automotive, 3D printing | [12] |
Overall, biopolymers offer promising steps towards a more sustainable future. Their natural origins, functional diversity, and biodegradability make them a valuable alternative to petrochemical polymers. Additionally, the applications of biopolymers are wide-ranging, including textiles, packaging, films, medicine, and agriculture. By understanding their unique properties and characteristics, it will be possible to use biopolymers to address environmental challenges and advance toward more sustainable materials production.
References
[1] Nair, N. R., et al. “Biodegradation of biopolymers.” Current developments in biotechnology and bioengineering. Elsevier, 2017. 739-755.
[2] Singh, Navodit Kumar, et al. “Application of plant products in the synthesis and functionalisation of biopolymers.” International Journal of Biological Macromolecules 237 (2023): 124174.
[3] Baranwal, Jaya, et al. “Biopolymer: A sustainable material for food and medical applications.” Polymers 14.5 (2022): 983.
[4] Crini, Grégorio. “Historical review on chitin and chitosan biopolymers.” Environmental Chemistry Letters 17.4 (2019): 1623-1643.
[5] Sun, Yitao, et al. “Global trends in natural biopolymers in the 21st century: a scientometric review.” Frontiers in Chemistry 10 (2022): 915648.
[6] Ling, Shengjie, et al. “Biopolymer nanofibrils: Structure, modeling, preparation, and applications.” Progress in polymer science 85 (2018): 1-56.
[7] Abdel-Rahman, Mohamed Ali, Yukihiro Tashiro, and Kenji Sonomoto. “Recent advances in lactic acid production by microbial fermentation processes.” Biotechnology advances 31.6 (2013): 877-902.
[8] Jamshidian, Majid, et al. “Poly‐lactic acid: production, applications, nanocomposites, and release studies.” Comprehensive reviews in food science and food safety 9.5 (2010): 552-571.
[9] Gordon, Stuart, and You-lo Hsieh, eds. Cotton: Science and technology. Woodhead Publishing, 2006.
[10] Moeller, Martin, and Krzysztof Matyjaszewski. Polymer science: a comprehensive reference. Newnes, 2012.
[11] Taib, Nur-Azzah Afifah Binti, et al. “A review on poly lactic acid (PLA) as a biodegradable polymer.” Polymer Bulletin 80.2 (2023): 1179-1213.
[12] Getahun, Muluken Jemberie, Bantamlak Birlie Kassie, and Tsega Samuel Alemu. “Recent advances in biopolymer synthesis, properties, & commercial applications: A review.” Process Biochemistry (2024).
[13] Younes, Islem, and Marguerite Rinaudo. “Chitin and chitosan preparation from marine sources. Structure, properties and applications.” Marine drugs 13.3 (2015): 1133-1174.
[14] Fazli Wan Nawawi, Wan Mohd, et al. “Chitin nanopaper from mushroom extract: natural composite of nanofibers and glucan from a single biobased source.” ACS Sustainable Chemistry & Engineering 7.7 (2019): 6492-6496.