19 Chapter 19 – Aldehydes and Ketones: Nucleophilic Addition Reactions Solutions to Problems
19.1 |
Remember that the principal chain must contain the aldehyde or ketone group and that an aldehyde group occurs only at the end of a chain. The aldehyde carbon is carbon 1 in an acyclic compound, and the suffix –carbaldehyde is used when the aldehyde group is attached to a ring. |
|
(a) |
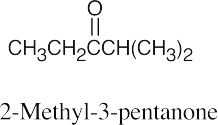 |
(b) |
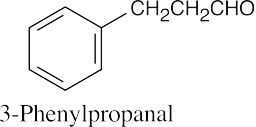 |
|
(c) |
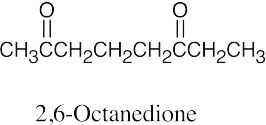 |
(d) |
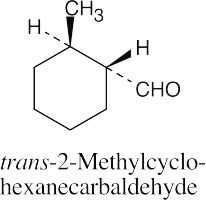 |
|
(e) |
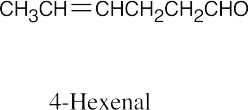 |
(f) |
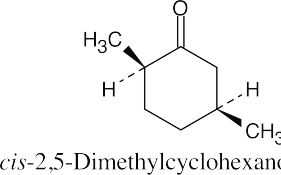 |
19.2 |
(a) |
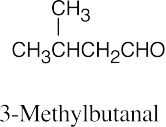 |
(b) |
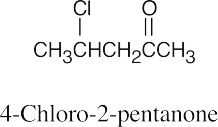 |
|
(c) |
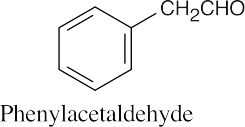 |
(d) |
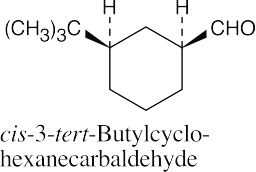 |
|
(e) |
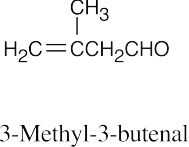 |
(f) |
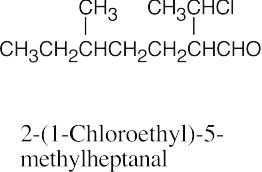 |
19.3 |
We have seen the first two methods of aldehyde preparation in earlier chapters. |
|
(a) |
 |
|
(b) |
 |
|
(c) |
 |
|
(d) |
 |
19.4 |
All of these methods are familiar. |
|
(a) |
 |
|
(b) |
 |
|
(c) |
 |
|
(d) |
 |
19.5 |
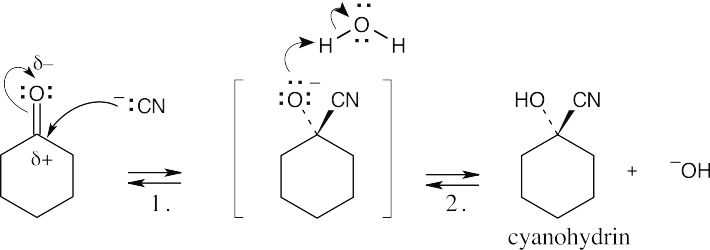 |
|
Step 1: |
Cyanide anion adds to the positively polarized carbonyl carbon to form a tetrahedral intermediate. |
|
Step 2: |
This intermediate is protonated to yield the cyanohydrin. |
19.6 |
The electron-withdrawing nitro group makes the aldehyde carbon of p-nitrobenzaldehyde more electron-poor (more electrophilic) and more reactive toward nucleophiles than the aldehyde carbon of p–methoxybenzaldehyde.
|
19.7 |
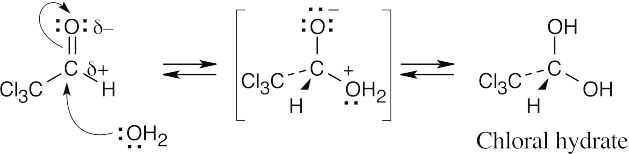 |
19.8 |
The above mechanism is similar to other nucleophilic addition mechanisms we have studied. Since all steps are reversible, we can write the above mechanism in reverse to show how labeled oxygen is incorporated into an aldehyde or ketone.

This exchange is very slow in water but proceeds more rapidly when either acid or base is present.
|
19.9 |
Cyanohydrin formation is an equilibrium process. Because addition of –CN to 2,2,6-trimethylcyclohexanone is sterically hindered by the three methyl groups, the equilibrium lies toward the side of the unreacted ketone.
|
19.10 |
Reaction of a ketone or aldehyde with a primary amine yields an imine, in which C=O has been replaced by C=NR. Reaction of a ketone or aldehyde with a secondary amine yields an enamine, in which C=O has been replaced by C–NR2, and the double bond has moved.
|
19.11 |
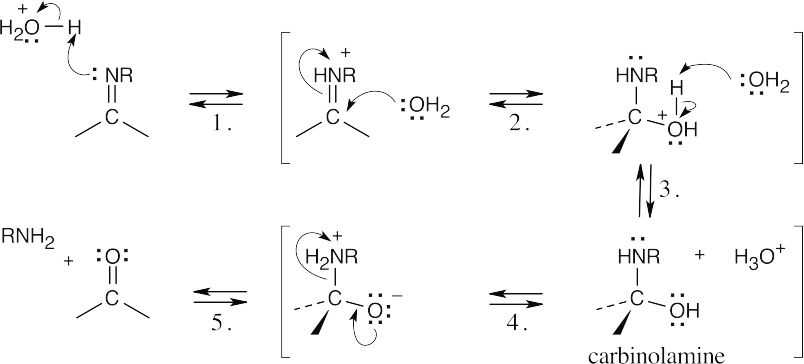 |
|
Step 1: |
Protonation of nitrogen. |
|
Step 2: |
Addition of water. |
|
Step 3: |
Loss of proton. |
|
Step 4: |
Proton transfer. |
|
Step 5: |
Loss of amine. |
19.12 |
The structure is an enamine, which is prepared from a ketone and a secondary amine. Find the amine and ketone components and draw the reaction.
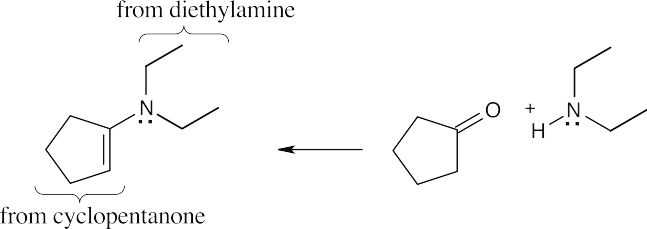
|
19.13 |
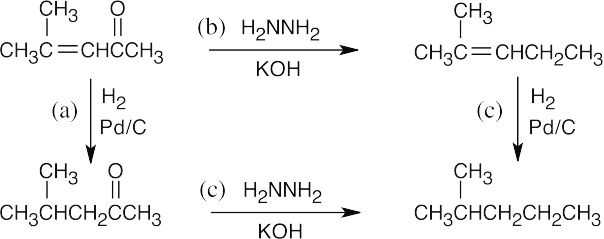 |
19.14 |
(a) |
Formation of the hemiacetal is the first step. |
|
|
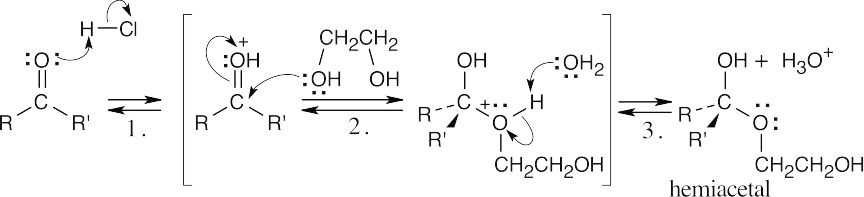 |
|
|
Step 1: |
Protonation of oxygen. |
|
|
Step 2: |
Addition of –OH. |
|
|
Step 3: |
Loss of proton. |
|
(b) |
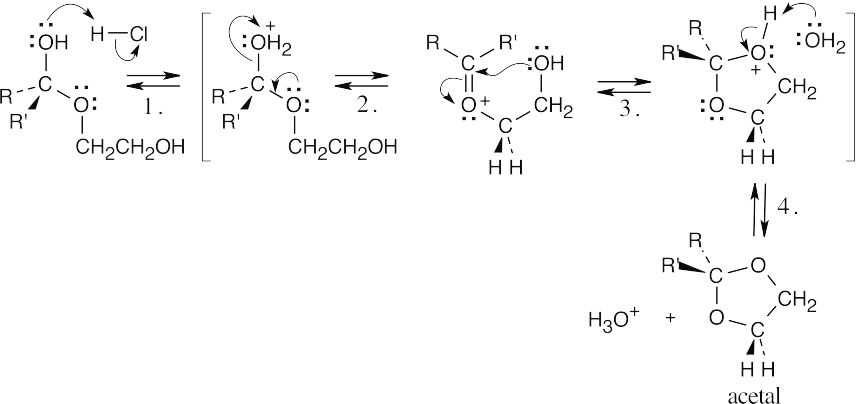 |
|
|
Step 1: |
Protonation. |
|
|
Step 2: |
Loss of H2O. |
|
|
Step 3: |
Addition of –OH. |
|
|
Step 4: |
Loss of proton. |
|
|
Protonation of the hemiacetal hydroxyl group is followed by loss of water. Attack by the second hydroxyl group of ethylene glycol forms the cyclic acetal ring. |
19.15 |
Locate the two identical –OR groups to identify the alcohol that was used to form the acetal. (The illustrated acetal was formed from methanol.) Replace these two –OR groups by =O to find the carbonyl compound.
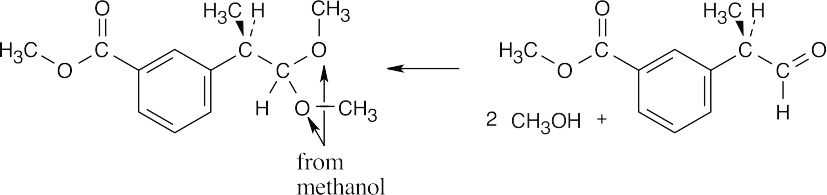
|
19.17 |
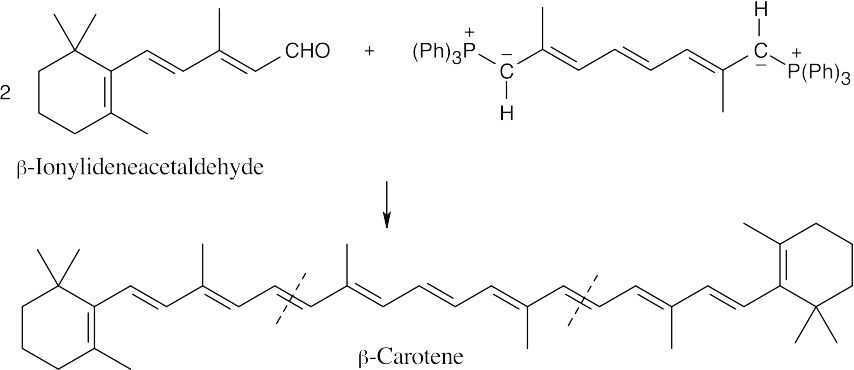 |
19.18 |
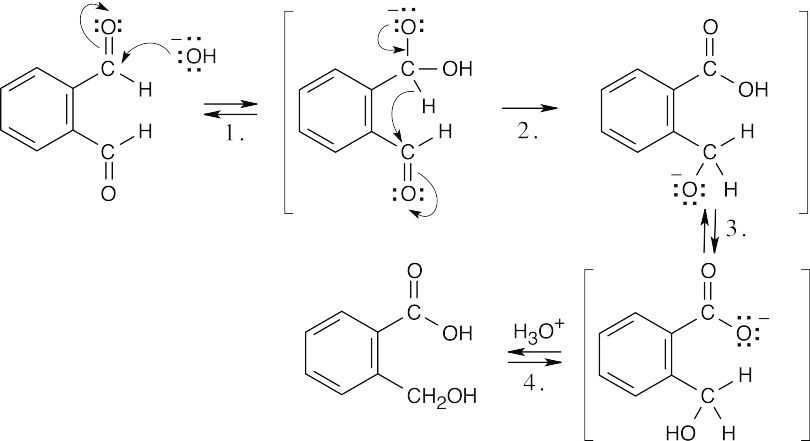 |
|
Step 1: |
Addition of –OH. |
|
Step 2: |
Expulsion, addition of –H. |
|
Step 3: |
Proton transfer. |
|
Step 4: |
Protonation. |
|
This is an intramolecular Cannizzaro reaction. |
19.19 |
Addition of the pro–R hydrogen of NADH takes place at the Re face of pyruvate.
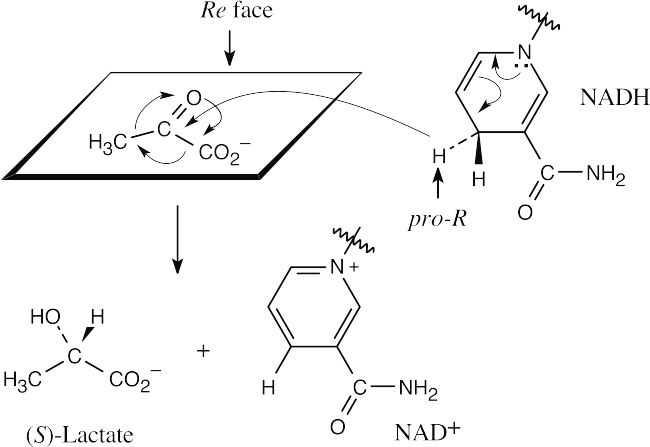
|
19.23 |
2-Cyclohexenone is a cyclic α,β-unsaturated ketone whose carbonyl IR absorption occurs at 1685 cm–1. If direct addition product A is formed, the carbonyl absorption vanishes and a hydroxyl absorption appears at 3300 cm–1. If conjugate addition produces B, the carbonyl absorption shifts to 1715 cm–1, where 6-membered-ring saturated ketones absorb. |
19.24 |
Find the type of aldehyde or ketone and check Table 19.2 for absorptions. |
|
(a) |
H2C=CHCH2COCH3 absorbs at 1715 cm–1. (4-Penten-2-one is not an α,β-unsaturated ketone.) |
|
(b) |
CH3CH=CHCOCH3 is an α,β-unsaturated ketone and absorbs at 1685 cm–1.
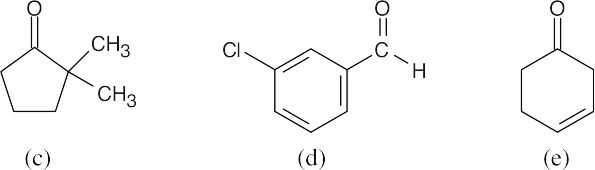
|
|
(c) |
2,2-Dimethylcyclopentanone, a five-membered-ring ketone, absorbs at 1750 cm–1. |
|
(d) |
m-Chlorobenzaldehyde shows an absorption at 1705 cm–1 and two absorptions at 2720 cm–1 and 2820 cm–1. |
|
(e) |
3-Cyclohexenone absorbs at 1715 cm–1. |
|
(f) |
CH3CH2CH2CH=CHCHO is an α,β-unsaturated aldehyde and absorbs at 1705 cm–1. |
19.25 |
In mass spectra, only charged particles are detected. The McLafferty rearrangement produces an uncharged alkene (not detected) and an oxygen-containing fragment, which is a cation radical and is detected. Alpha cleavage produces a neutral radical (not detected) and an oxygen-containing cation, which is detected. Since alpha cleavage occurs primarily on the more substituted side of the aldehyde or ketone, only this cleavage is shown. |
|
(a) |
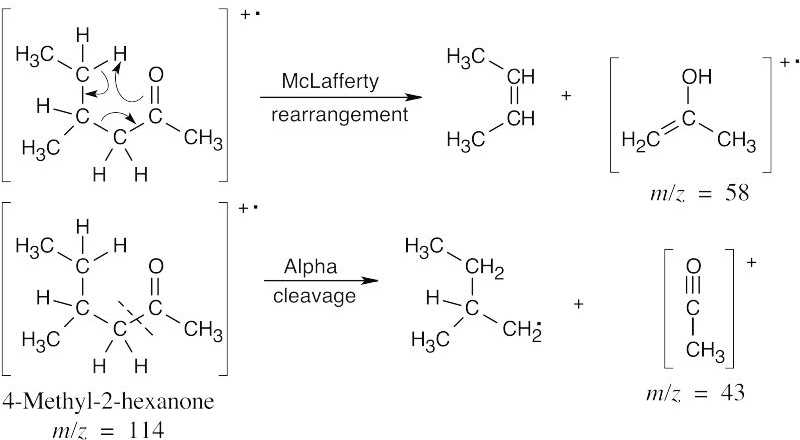
Both isomers exhibit peaks at m/z = 43 due to α-cleavage. The products of McLafferty rearrangement, however, occur at different values of m/z and can be used to identify each isomer.
|
|
(b) |
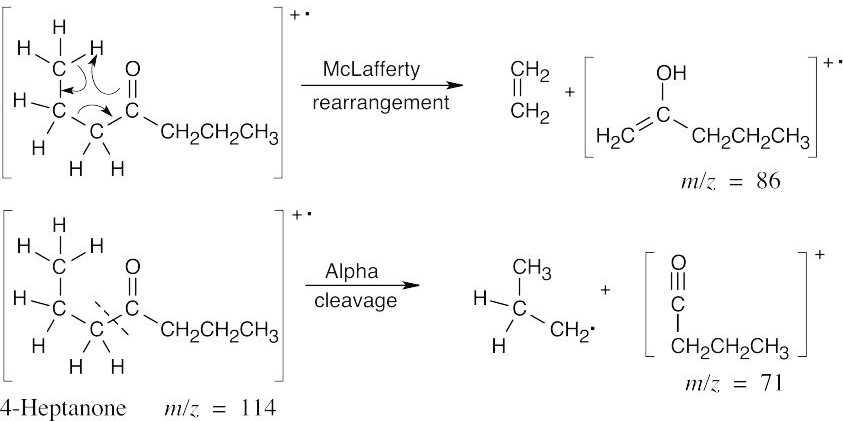
The isomers can be distinguished on the basis of both α-cleavage products (m/z = 57 vs m/z = 71) and McLafferty rearrangement products (m/z = 72 vs m/z = 86). |
|
(c) |
The fragments from McLafferty rearrangement, which occur at different values of m/z, serve to distinguish the two isomers.
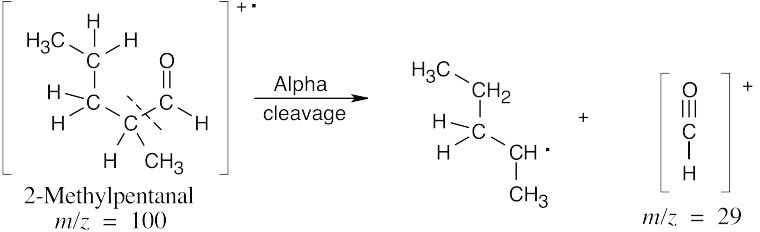
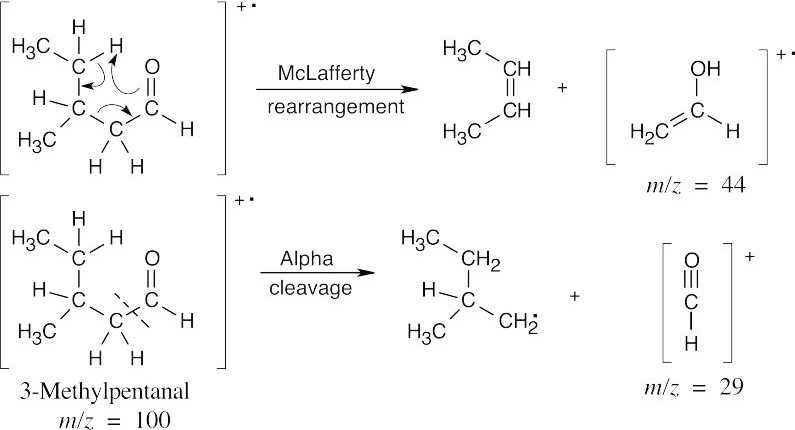
|
19.26 |
IR: The only important IR absorption for the compound is seen at 1750 cm–1, where 5-membered ring ketones absorb. |
|
Mass spectrum: The products of alpha cleavage, which occurs in the ring, have the same mass as the molecular ion.
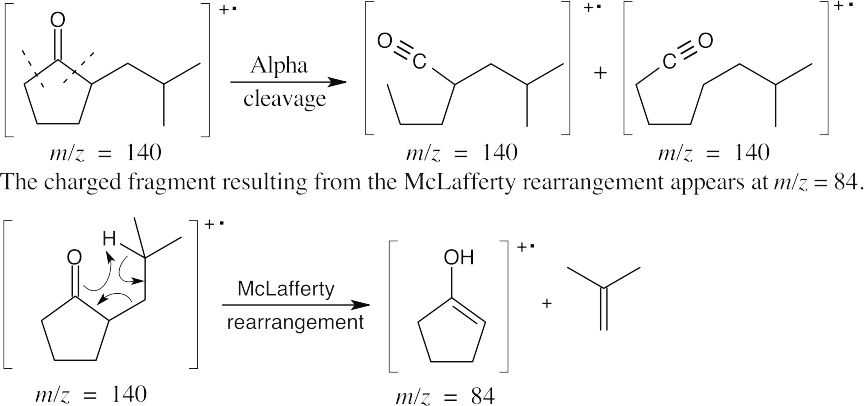
|
Additional Problems
Visualizing Chemistry
19.27 |
It helps to know that all of these substances were prepared from aldehydes or ketones. Look for familiar groupings of atoms to identify the starting materials. |
|
(a) |
Notice that the substance pictured is a cyclic acetal. The starting materials were a diol (because cyclic acetals are prepared from diols) and an aldehyde (because an –H is bonded to the acetal carbon). Replace the two –OR groups with =O to identify the aldehyde starting material (acetaldehyde).

|
|
(b) |
We know that the product is an imine because it contains a carbon-nitrogen double bond. The carbon that is part of the C=N bond came from a ketone, and the nitrogen came from a primary amine.
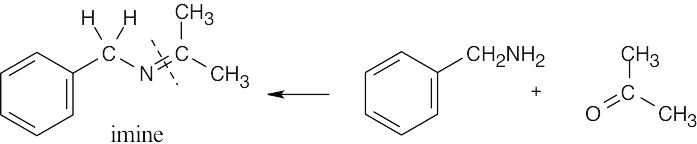
|
|
(c) |
The product is an enamine, formed from a ketone and a secondary amine. Nitrogen is bonded to the carbon that once bore the carbonyl oxygen.

|
|
(d) |
The secondary alcohol product might have been formed by either of two routes – by reduction of a ketone or by Grignard addition to an aldehyde.
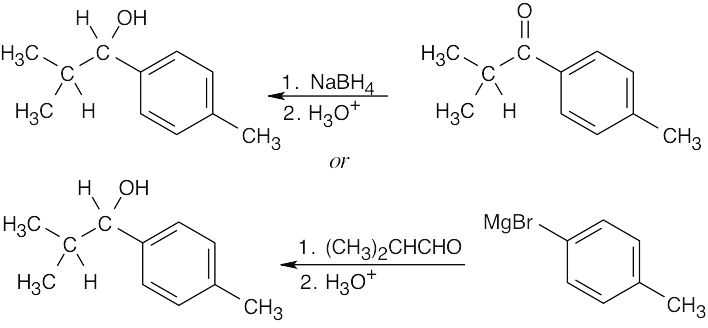
|
19.28 |
The intermediate results from the addition of an amine to a ketone. The product is an enamine because the amine nitrogen in the carbinolamine intermediate comes from a secondary amine.

|
19.29 |
(a) |
The nitrogen atom is sp2-hybridized, and the geometry is planar. |
|
(b) |
A p orbital holds the lone-pair electrons of nitrogen. |
|
(c) |
The p orbital holding the lone-pair electrons of nitrogen is aligned for overlap with the π electrons of the enamine double bond. With this geometry, the nitrogen lone-pair electrons can be conjugated with the double bond, thus lowering energy.
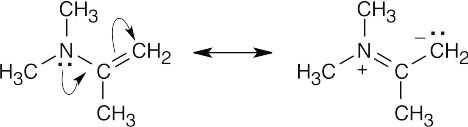
|
Mechanism Problems
19.30 |
Note: While there may be several resonance forms for a particular intermediate, these solutions will always use the most stable form. |
|
(a) |
Mechanism:

|
|
(b) |
Mechanism:

and

|
19.31 |
Note: Each reaction is an example of acetal formation and follows the same pattern: 1) protonation of the carbonyl oxygen; 2) nucleophilic addition of an alcohol oxygen; 3) removal of a proton from the oxygen now bearing the positive charge to give a hemiacetal intermediate. This is often accomplished with the solvent. In the problems where no solvent is given, a general base has been used for these solutions; 4) protonation of the hemiacetal OH converting it to water, a good leaving group; 5) loss of water to form a highly electrophilic oxonium ion; 6) nucleophilic addition of a second alcohol oxygen to the carbon adjacent to the oxonium ion; 7) deprotonation to yield the acetal product. |
|
(a) |
Mechanism:
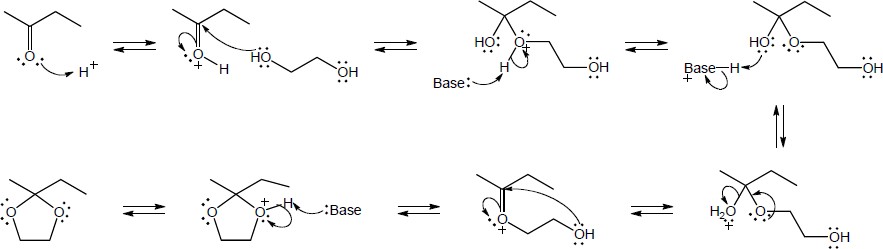
|
|
(b) |
Mechanism:
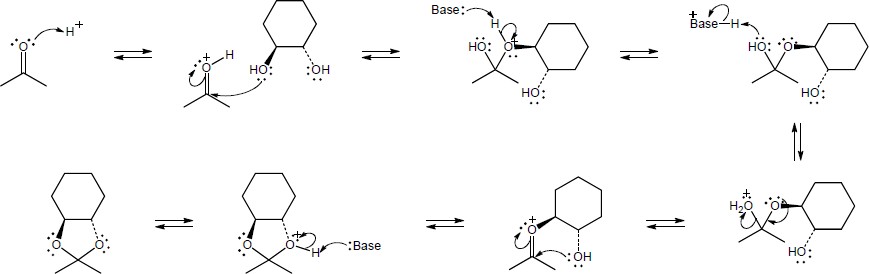
|
19.32 |
Note: Each mechanism is the acid catalyzed hydrolysis of an acetal and follows the same steps. Fundamentally, acetal hydrolysis is the mechanism for acetal formation in reverse. The steps are: 1) Protonation of one of the acetal oxygens; 2) Loss of an alcohol molecule with simultaneous formation of an oxonium ion; 3) Nucleophilic addition of water to the carbon that is double bonded to the oxonium ion; 4) Deprotonation of the oxygen with the positive charge to give a hemiacetal intermediate; 5) Protonation of the ether oxygen of the hemiacetal; 6) Loss of a second alcohol molecule with simultaneous formation of carbon-oxygen double double bond; 7) Deprotonation of the carbonyl oxygen to give the carbonyl. |
|
(a) |
Mechanism:
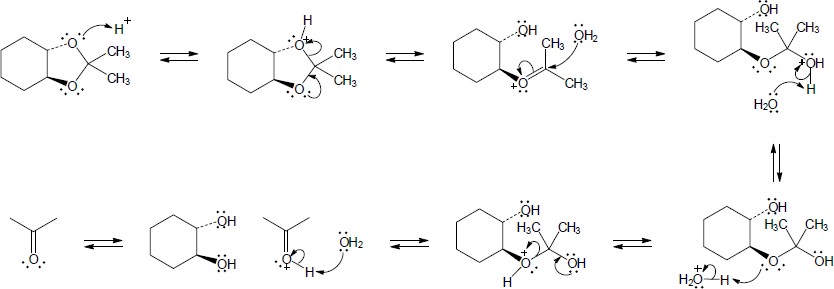
|
|
(b) |
Mechanism:
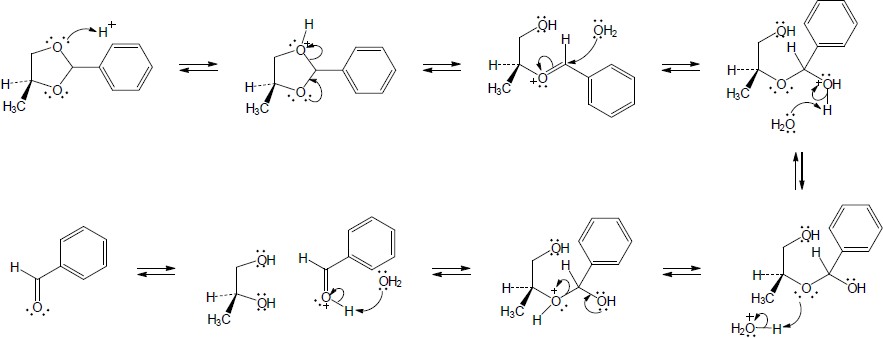
|
19.33 |
Note: Each mechanism involves the following steps for iminium ion formation: 1) Nucleophilic addition of the amine nitrogen to the carbonyl carbon to form a dipolar tetrahedral intermediate; 2) Proton transfer to yield a neutral carbonolamine; 3) Protonation of the OH group of the carbonolamine to convert it into a water, a good leaving group; 4) Loss of water through use of the lone pair of electrons on the nitrogen of the carbinolamine to form an iminium ion. At this point, the mechanism differs: if the amine is primary, the iminium ion is deprotonated to yield an imine; if the amine is secondary, an alpha hydrogen is removed in an E2-like process to yield an enamine. |
|
(a) |
Mechanism:
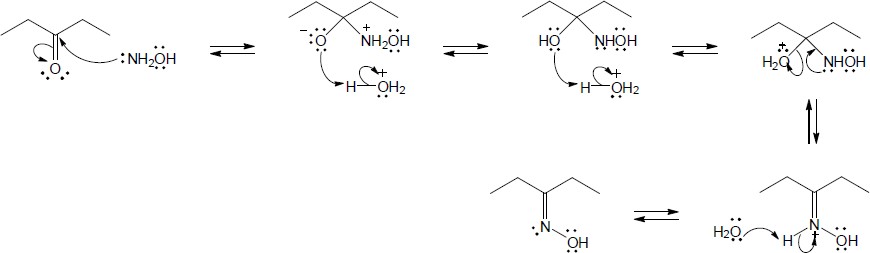
|
|
(b) |
Mechanism:
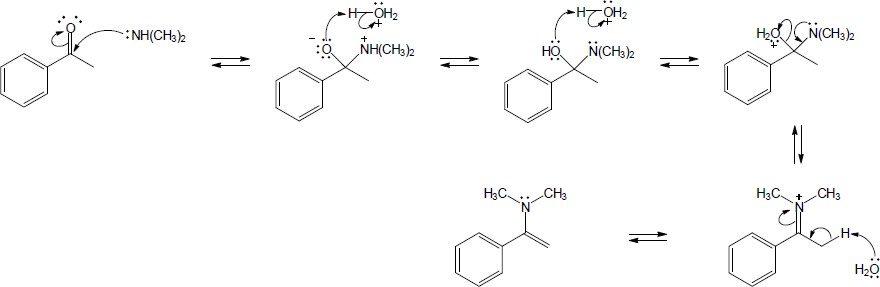
|
19.34 |
Note: Each mechanism is an example of imine or enamine hydrolysis. The entire mechanism for each is the same with the exception of the first step. In problem (a), the first step is protonation of the imine nitrogen to give an iminium ion. In problem (b), the first step is protonation of the b-carbon. The remaining steps, which are identical for both mechanisms, follow: 2) Nucleophilic addition of water to the iminium carbon; 3) Deprotonation of the positively charged oxygen to form an intermediate carbinolamine; 4) Protonation of the carbinolamine nitrogen; 5) Expulsion of a neutral amine with simultaneous formation of a carbonyl; 6) Deprotonation of the carbonyl and protonation of the free amine. |
|
(a) |
Mechanism:
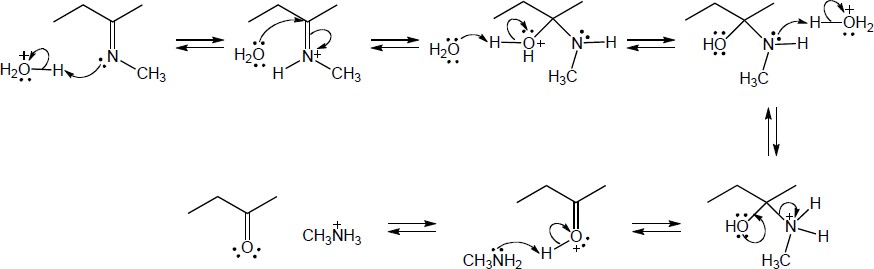
|
|
(b) |
Mechanism:
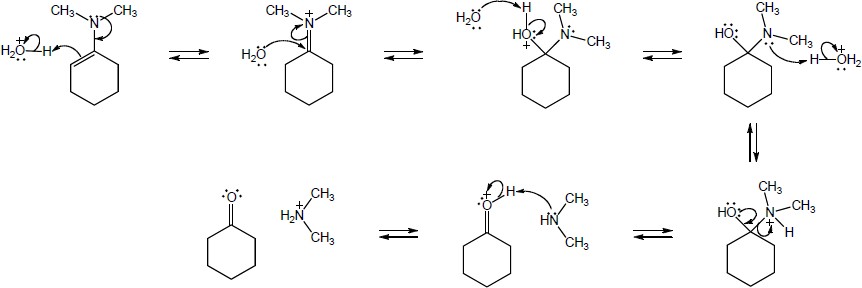
|
19.35 |
(a) |
Mechanism:
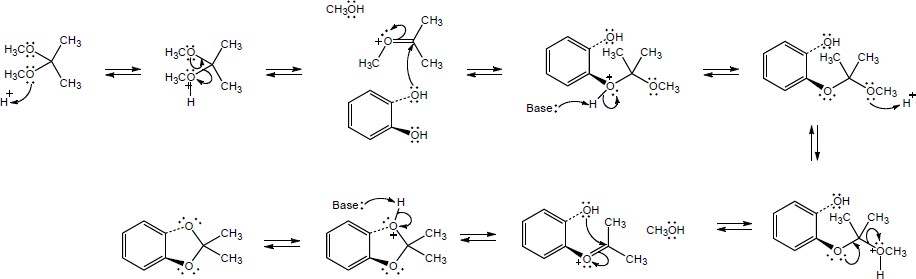
|
|
(b) |
Mechanism:
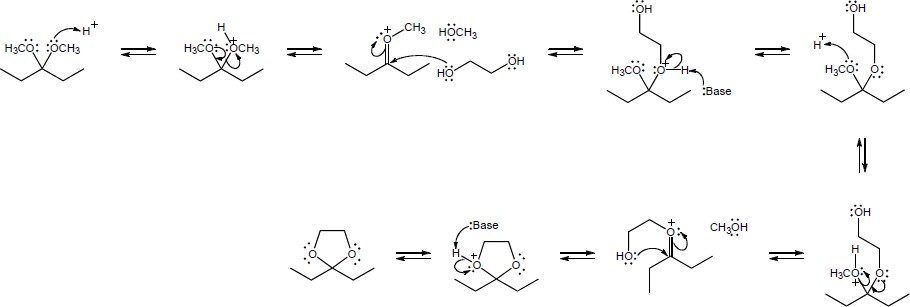
|
19.36 |
Mechanism:
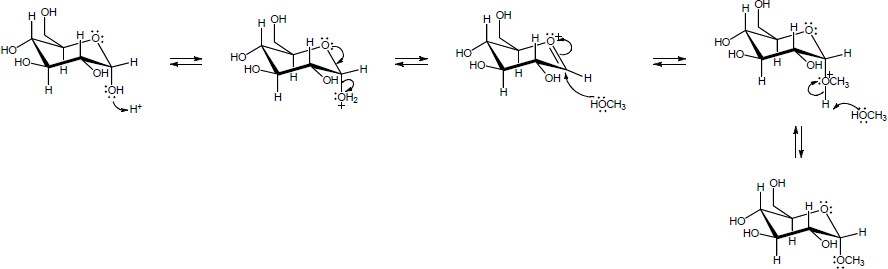
|
19.37 |
(a) |
Mechanism:
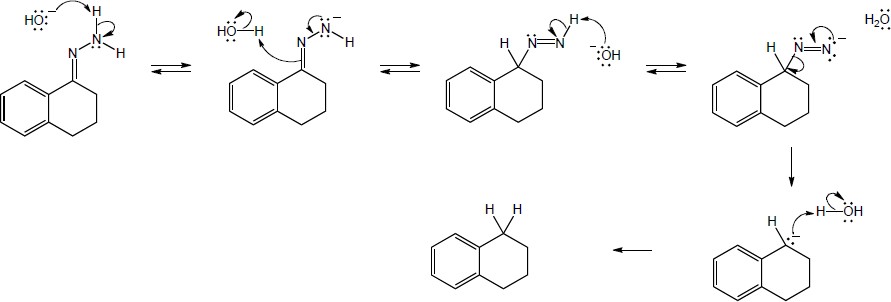
|
|
(b) |
Mechanism:
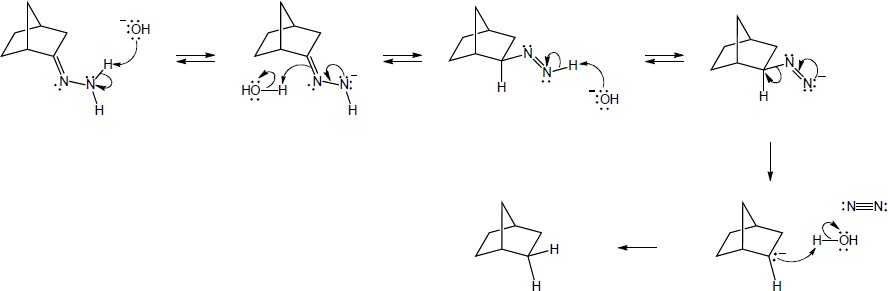
|
19.38 |
(a) |
 |
|
(b) |
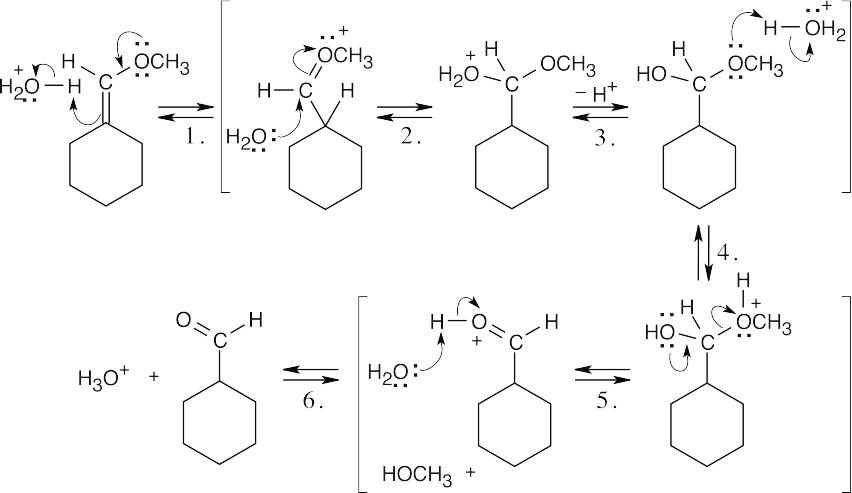 |
|
Step 1, 4: |
Protonation. |
|
Step 2: |
Addition of water. |
|
Step 3, 6: |
Deprotonation. |
|
Step 4: |
Loss of CH3OH. |
19.39 |
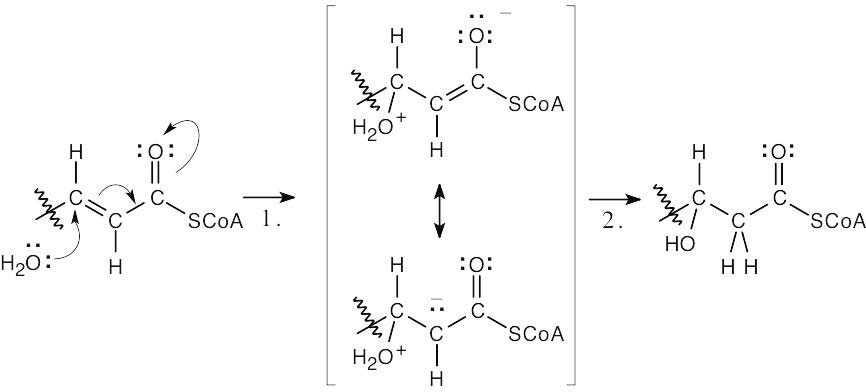 |
|
Step 1: |
Conjugate addition of water. |
|
Step 2: |
Proton shift. |
19.40 |
The same series of steps used to form an acetal is followed in this mechanism.
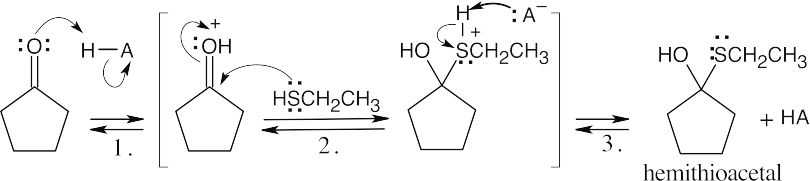
|
|
Step 1: |
Protonation. |
|
Step 2: |
Addition of RSH. |
|
Step 3: |
Loss of proton. |
|
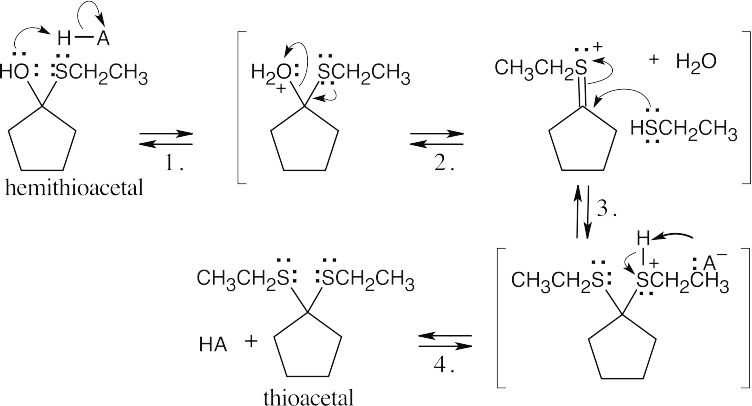 |
|
Step 1: |
Protonation. |
|
Step 2: |
Loss of water. |
|
Step 3: |
Addition of RSH. |
|
Step 4:
|
Loss of proton. |
19.41 |
Even though the product looks unusual, this reaction is made up of steps with which you are familiar.

|
|
Step 1: |
Addition of ylide. |
|
Step 2: |
SN2 displacement of dimethyl sulfide by O–. |
19.42 |
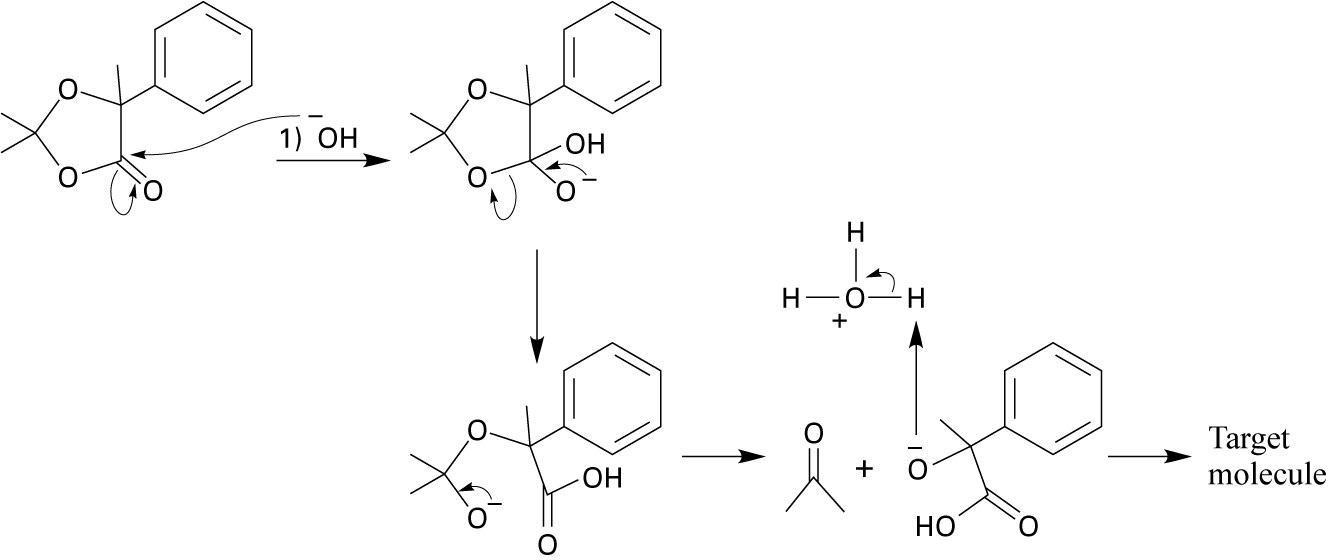 |
19.43 |
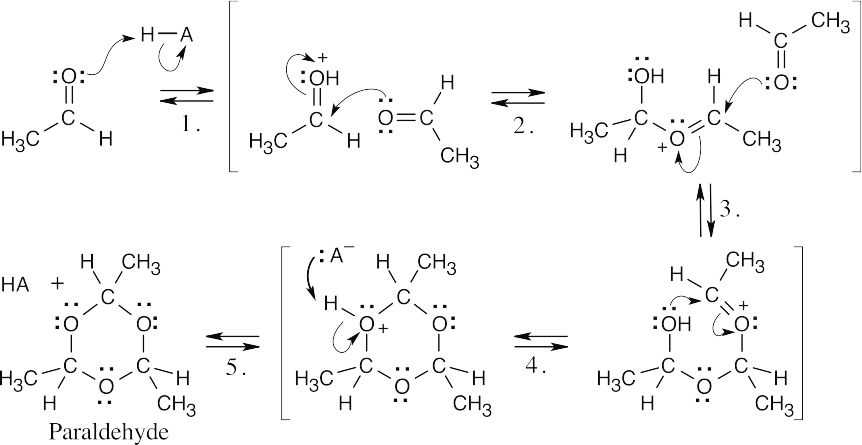 |
|
Step 1: |
Protonation makes the carbonyl carbon more electrophilic. |
|
Step 2, 3, 4: |
Three successive additions of the carbonyl oxygen of acetaldehyde to the electrophilic carbonyl carbon, followed by loss of a proton (Step 5), give the cyclic product. |
19.44 |
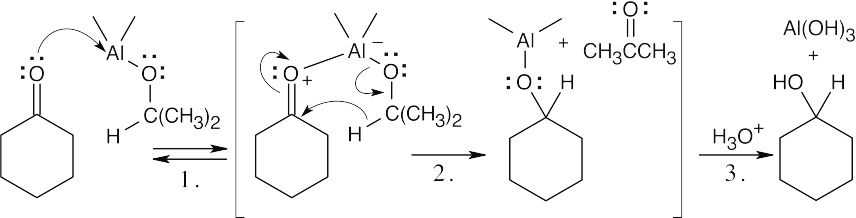 |
|
Step 1: |
Aluminum, a Lewis acid, complexes with the carbonyl oxygen. |
|
Step 2: |
Complexation with aluminum makes the carbonyl group more electrophilic and facilitates hydride transfer from isopropoxide. |
|
Step 3: |
Treatment of the reaction mixture with aqueous acid cleaves the aluminum–oxygen bond and produces cyclohexanol. |
|
Both the Meerwein–Ponndorf–Verley reaction and the Cannizzaro reaction are hydride transfers in which a carbonyl group is reduced by an alkoxide group, which is oxidized. Note that each aluminum triisopropoxide molecule is capable of reducing three ketone molecules. |
19.45 |
(a) |
Nucleophilic addition of one nitrogen of hydrazine to one of the carbonyl groups, followed by elimination of water, produces a hydrazone.
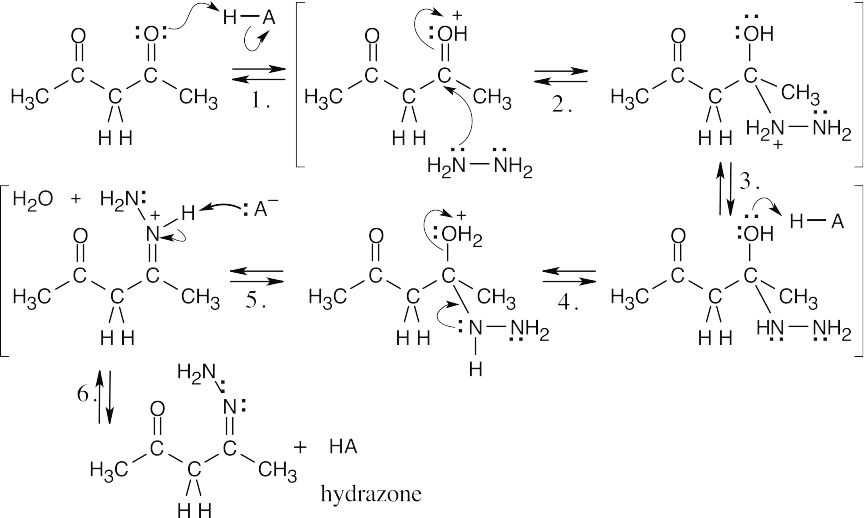
|
|
(b) |
In a similar manner, the other nitrogen of hydrazine can add to the other carbonyl group of 2,4-pentanedione to form the pyrazole.
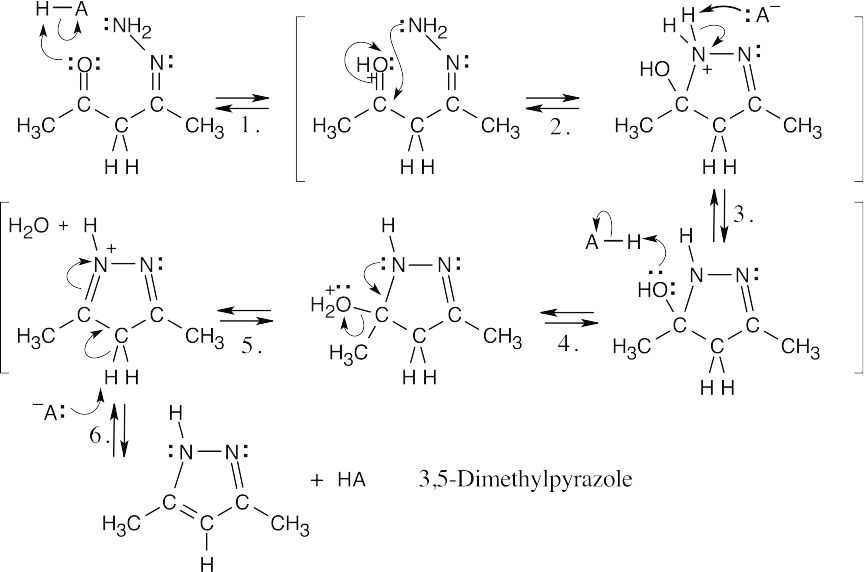
The driving force behind this reaction is the formation of an aromatic ring. The reactions in both parts of this problem are nucleophilic addition of a primary amine (Step 2), followed by elimination of water to yield an imine or enamine (Step 5). All of the other steps are protonations (Steps 1,4) and deprotonations (Steps 3,6). |
19.46 |
The same sequence of steps used in the previous problem leads to the formation of 3,5-dimethylisoxazole when hydroxylamine is the reagent. Loss of a proton in the last step of (b) results in a ring that is aromatic. |
|
(a) |
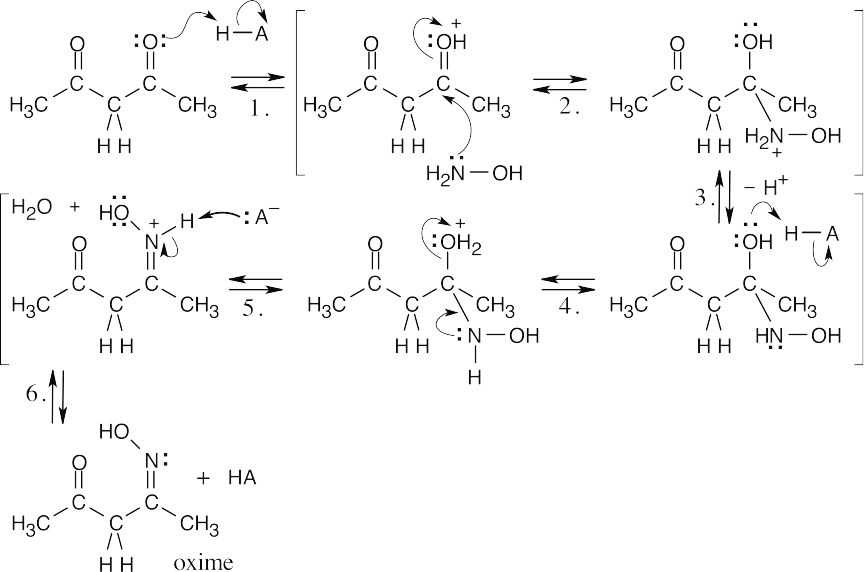 |
|
(b) |
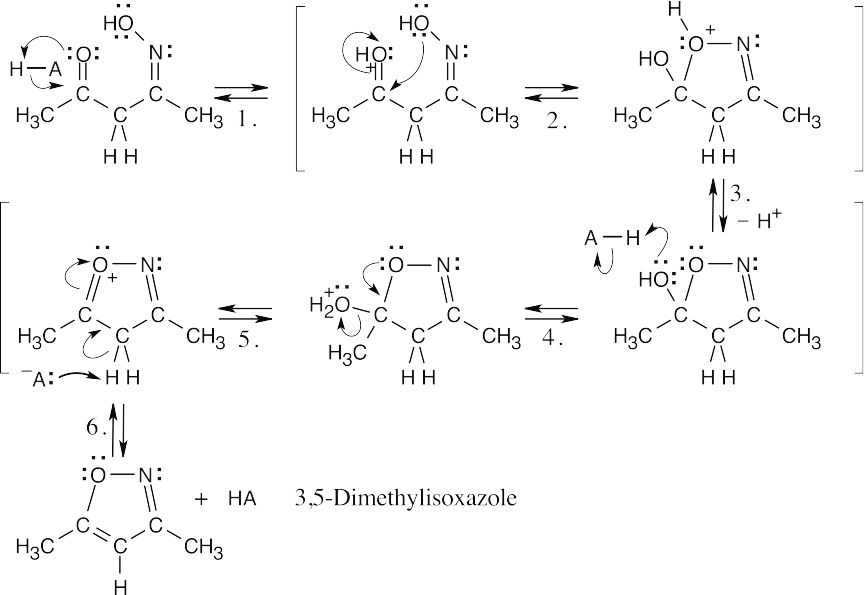 |
19.47 |
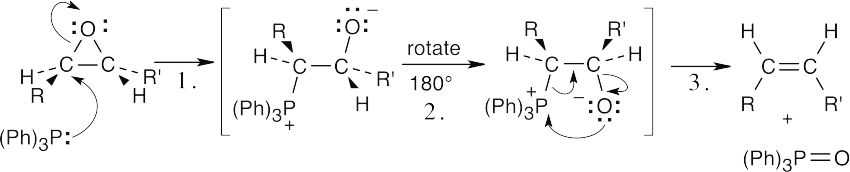 |
|
Step 1: |
Addition of the phosphine nucleophile. |
|
Step 2: |
Rotation of C–C bond. |
|
Step 3: |
Elimination of triphenylphosphine oxide. |
|
The final step is the same as the last step in a Wittig reaction. The same series of steps converts a trans alkene to a cis alkene. |
19.48 |
Hydrogen peroxide and hydroxide react to form water and hydroperoxide anion.

Conjugate addition of hydroperoxide anion (Step 1) is followed by elimination of hydroxide ion, with formation of the epoxide ring (Step 2).
|
19.49 |
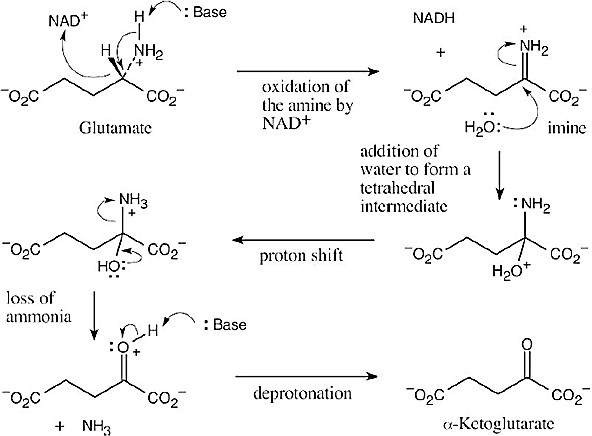
|
19.50 |
In this series of reactions, conjugate addition of an amine to an α,β-unsaturated ester (Step 1) is followed by nucleophilic addition of the amine to a second ester (Step 3), with loss of methanol (Step 5). The other two steps are proton transfers.
|
19.51 |
Some hydrogens have been omitted to make the drawing less cluttered.
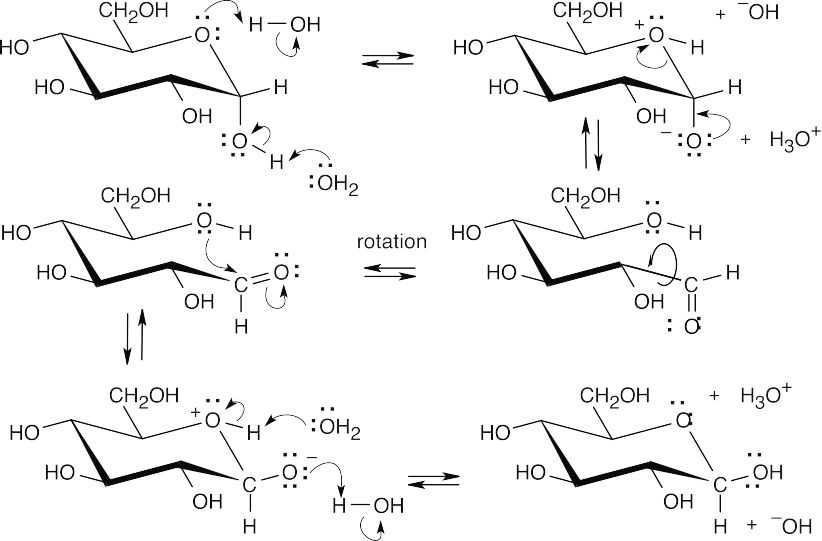
In this series of equilibrium steps, the hemiacetal ring of α-glucose opens to yield the free aldehyde. Bond rotation is followed by formation of the cyclic hemiacetal of ß-glucose. The reaction is catalyzed by both acid and base.
|
19.54 |
Only 2-methylbutanal is chiral.
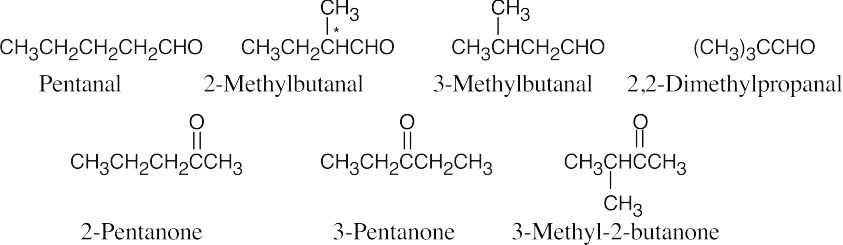
|
19.55 |
(a) |
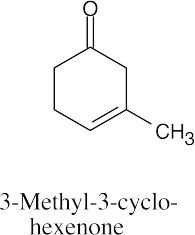 |
(b) |
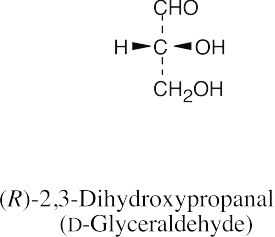 |
(c) |
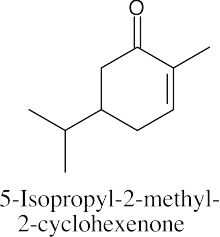
|
|
(d) |
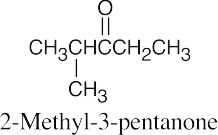 |
(e) |
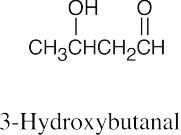 |
(f) |
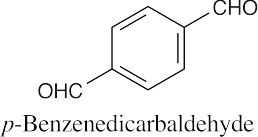 |
19.56 |
(a) |
The α,β-unsaturated ketone C6H8O contains one ring. Possible structures include:
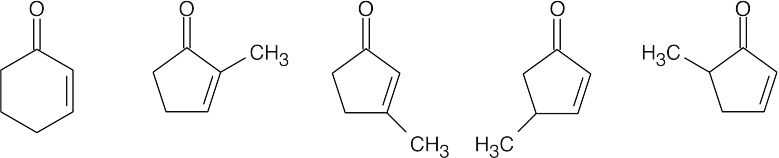
Cyclobutenones and cyclopropenones are also possible.
|
|
(b) |
 |
|
(c) |
 |
|
(d) |
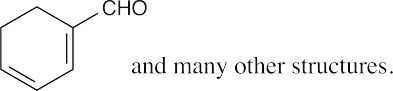 |
Reactions of Aldehydes and Ketones
19.57 |
Reactions of phenylacetaldehyde: |
|
(a) |
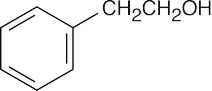 |
(b) |
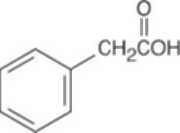 |
(c) |
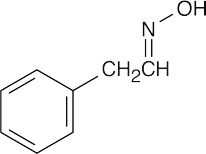 |
|
(d) |
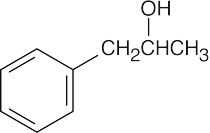 |
(e) |
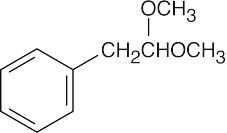 |
(f) |
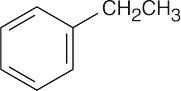 |
|
(g) |
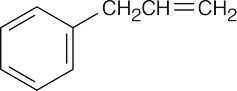 |
(h) |
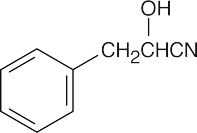 |
|
|
|
Reactions of acetophenone: |
|
(a) |
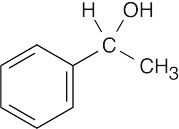 |
(b) |
No reaction |
(c) |
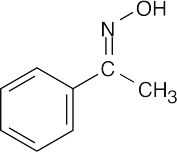 |
|
(d) |
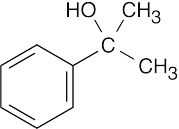 |
(e) |
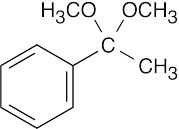 |
(f) |
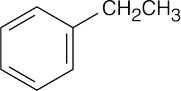 |
|
(g) |
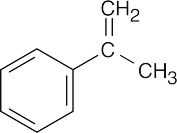 |
(h) |
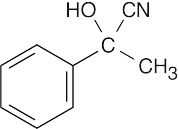 |
|
|
19.60 |
In general, ketones are less reactive than aldehydes for both steric (excess crowding) and electronic reasons. If the keto aldehyde in this problem were reduced with one equivalent of NaBH4, the aldehyde functional group would be reduced in preference to the ketone.
For the same reason, reaction of the keto aldehyde with one equivalent of ethylene glycol selectively forms the acetal of the aldehyde functional group. The ketone can then be reduced with NaBH4 and the acetal protecting group can be removed.
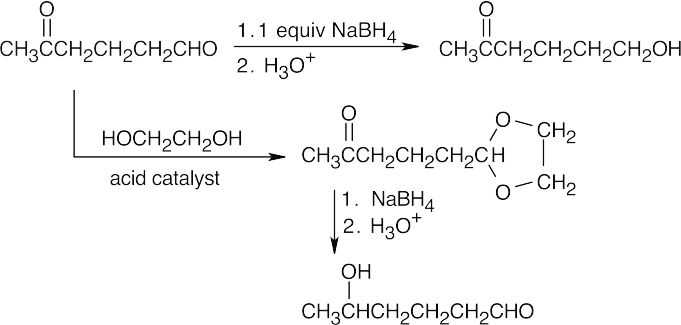
|
19.62 |
(a) |
The product resembles the starting material in having an aldehyde group, but a – CH2– group lies between the aldehyde and the aromatic ring. The product aldehyde results from oxidation of an alcohol that is the product of a Grignard reaction between formaldehyde and benzylmagnesium bromide. The Grignard reagent is formed from benzyl bromide, which results from treatment of benzyl alcohol with PBr3. Reduction of benzaldehyde yields the alcohol.
An alternate route:
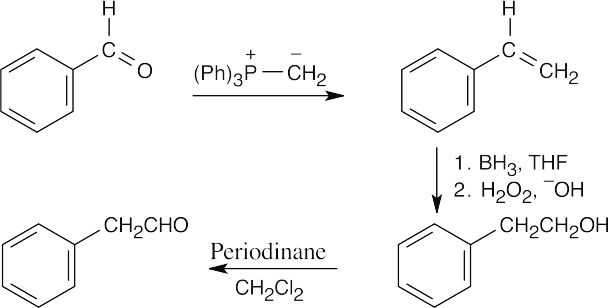
In this scheme, the intermediate alcohol results from hydroboration of a double bond that is introduced by a Wittig reaction between benzaldehyde and methylenetriphenylphosphorane.
|
|
(b) |
When you see a secondary amine and a double bond, you should recognize an enamine. The enamine is formed from the amine and acetophenone. Acetophenone, in turn, results from a reaction of benzaldehyde with methylmagnesium bromide, followed by oxidation.
|
|
(c) |
The trisubstituted double bond suggests a Wittig reaction. Reaction of cyclopentanone with the Wittig reagent formed from benzyl bromide (formed from benzaldehyde in (a)) yields the desired product.
|
19.63 |
(a) |
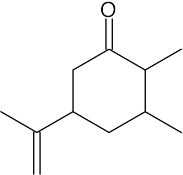 |
(b) |
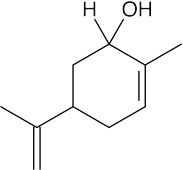 |
(c) |
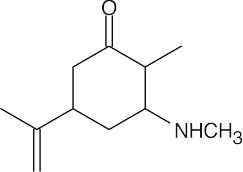 |
|
(d) |
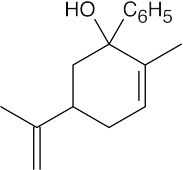 |
(e) |
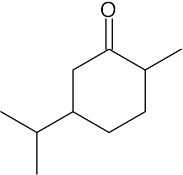 |
(f) |
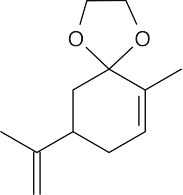 |
|
(g) |
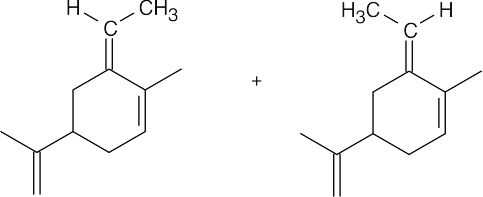 |
|
|
19.64 |
(a) |
The methyl group is introduced by a Grignard reaction with methylmagnesium bromide. Dehydration of the resulting tertiary alcohol produces 1-methylcyclohexene.
|
|
(b) |
Reaction with phenylmagnesium bromide yields a tertiary alcohol that can be dehydrated. The resulting double bond can be treated with BH3 to give an alcohol that can be oxidized to produce the desired ketone.
|
|
(c) |
Reduction, dehydration and hydroxylation yield the desired product.
|
|
(d) |
A Grignard reaction forms 1-cyclohexylcyclohexanol.
|
Spectroscopy
19.65 |
Use Table 19.2 if you need help. Only carbonyl absorptions are noted. |
|
|
Absorption: |
Due to: |
|
(a) |
1750 cm–1
1685 cm–1
|
5-membered ring ketone
α,ß-unsaturated ketone
|
|
(b) |
1720 cm–1 |
5-membered ring and aromatic ketone |
|
(c) |
1750 cm–1 |
5-membered ring ketone |
|
(d) |
1705 cm–1, 2720 cm–1,
2820 cm–1, 1715 cm–1 |
aromatic aldehyde
aliphatic ketone |
|
Compounds in parts (b)–(d) also show aromatic ring IR absorptions in the range 1450 cm–1–1600 cm–1 and in the range 690–900 cm–1.
|
19.66 |
Compound A is a cyclic, nonconjugated enone whose carbonyl infrared absorption should occur at 1715 cm–1. Compound B is an α,β-unsaturated, cyclic ketone; additional conjugation with the phenyl ring should lower its IR absorption below 1685 cm–1.
Because the actual IR absorption occurs at 1670 cm–1, B is the correct structure. |
19.67 |
undecylenic aldehyde |
19.75 |
(a) |
Grignard addition to a conjugated ketone yields the 1,2 product, not the 1,4 product. LiAlH4 reduces a ketone to an alcohol. The correct scheme:

|
|
(b) |
Oxidation of an alcohol with acidic CrO3 converts primary alcohols to carboxylic acids, not to aldehydes. The correct scheme:

|
|
(c) |
Treatment of a cyanohydrin with H3O+ produces a carboxylic acid, not an amine. The correct scheme:

|
19.76 |
The reaction sequence involves protecting the ketone, converting the ester to an aldehyde, using a Wittig reaction to introduce a substituted double bond, and deprotecting the ketone.
|
19.77 |
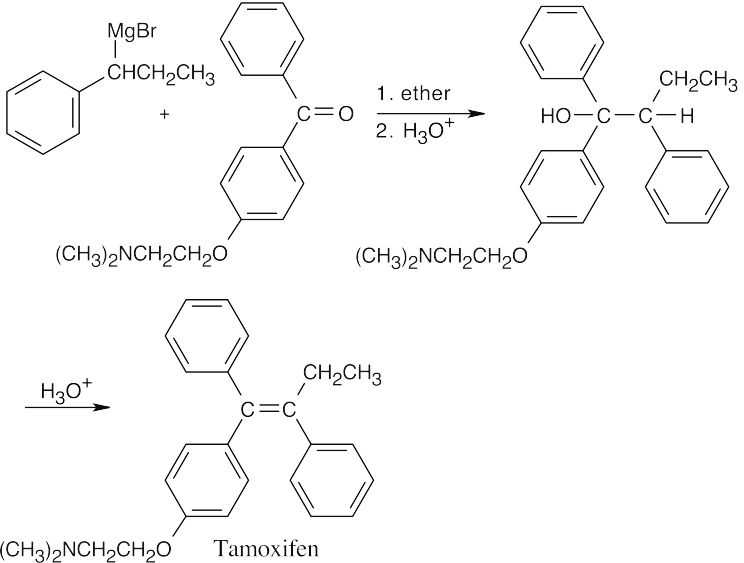
When you see a product that contains a double bond, and you also know that one of the starting materials is a ketone, it is tempting to use a Wittig reaction for synthesis. In this case, however, the tetrasubstituted double bond can’t be formed by a Wittig reaction because of steric hindrance. The coupling step is achieved by a Grignard reaction between the illustrated ketone and a Grignard reagent, followed by dehydration. The Grignard reagent is synthesized from 1-phenyl-1-propanol, which can be prepared from benzene by either of two routes.
|
19.78 |
The molecular weight of Compound A shows that the molecular formula of A is C5H10O (one degree of unsaturation), and the IR absorption shows that A is an aldehyde. The uncomplicated 1H NMR is that of 2,2-dimethylpropanal.
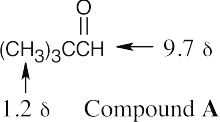
|
19.79 |
The IR of Compound B shows a ketone absorption. The splitting pattern of the 1H NMR spectrum indicates an isopropyl group and indicates that the compound is a methyl ketone.
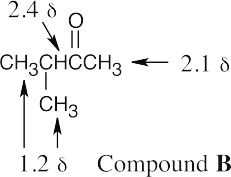
|
19.80 |
Before looking at the 1H NMR spectrum, we know that the compound of formula C9H10O has 5 degrees of unsaturation, and we know from the IR spectrum that the unknown is an aromatic ketone. The splitting pattern in the 1H NMR spectrum shows an ethyl group next to a ketone, according to chemical shift values.
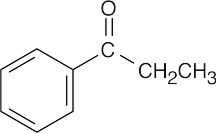
|
19.81 |
The IR absorption is that of an aldehyde that isn’t conjugated with the aromatic ring. The two triplets in the 1H NMR spectrum are due to two adjacent methylene groups.
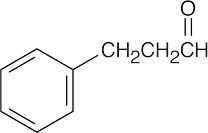
|
19.82 |
(a) |
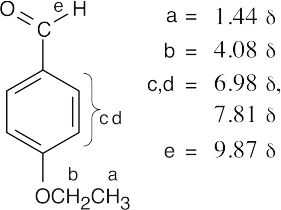 |
|
(b) |
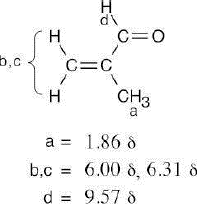 |
|
19.83 |
(a) |
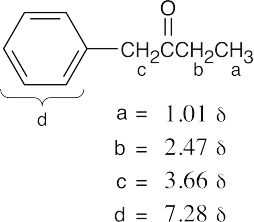 |
|
(b) |
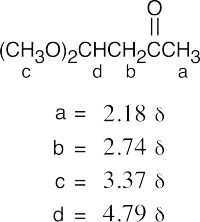 |
|
19.84 |
The free aldehyde form of glucose (Problem 19.72) is reduced in the same manner described in the text for other aldehydes to produce the polyalcohol sorbitol.
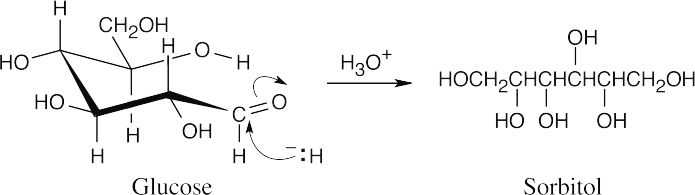
|
19.85 |
(a) |
4-Heptanone
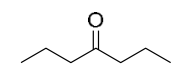
|
|
(b) |
2,4-Dimethyl-3-pentanone

|
|
(c) |
4,4-Dimethyl-2-pentanone
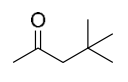
|
19.86 |
4-Methoxyphenylacetone |
This file is copyright 2023, Rice University. All Rights Reserved.