7 Chapter 7 – Alkenes: Structure and Reactivity Solutions to Problems
7.1 |
Because two hydrogens must be removed from a saturated compound to introduce an unsaturation, a compound’s degree of unsaturation refers to the number of pairs of hydrogens by which its formula differs from that of the corresponding saturated compound. For example, a saturated alkane with four carbons has the formula C4H10. The compound in (a), C4H8, which has two fewer (or one pair fewer) hydrogens, may have a double bond or a ring. C8H14 thus has a degree of unsaturation of 1.
Compound
|
Degree of Unsaturation
|
Structures
|
(a) C4H8
|
1
|

|
(b) C4H6
|
2
|
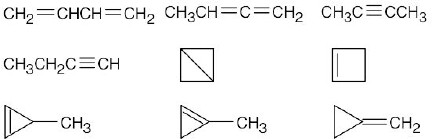
|
(c) C3H4
|
2
|

|
|
|
|
|
7.2 |
Unlike the hydrocarbons in the previous problems, the compounds in this problem contain additional elements. Review the rules for these elements. |
|
(a) |
Subtract one hydrogen for each nitrogen present to find the formula of the equivalent hydrocarbon –C6H4. Compared to the alkane C6H14, the compound of formula C6H4 has 10 fewer hydrogens, or 5 fewer hydrogen pairs, and has a degree of unsaturation of 5. |
|
(b) |
C6H5NO2 also has 5 degrees of unsaturation because oxygen does not affect the equivalent hydrocarbon formula of a compound. |
|
(c) |
A halogen atom is equivalent to a hydrogen atom in calculating the equivalent hydrocarbon formula. For C8H9Cl3, the equivalent hydrocarbon formula is C8H12, and the degree of unsaturation is 3. |
|
(d) |
C9H16Br2 – one degree of unsaturation. |
|
(e) |
C10H12N2O3 – 6 degrees of unsaturation. |
|
(f) |
C20H32ClN – 5 degrees of unsaturation. |
7.3 |
A C16 hydrocarbon with 11 degrees of unsaturation (three rings and eight double bonds) has a formula C16H34 – H22 = C16H12. Adding two hydrogens (because of the two nitrogens) and subtracting one hydrogen (because of the chlorine), gives the formula C16H13ClN2O for Diazepam.
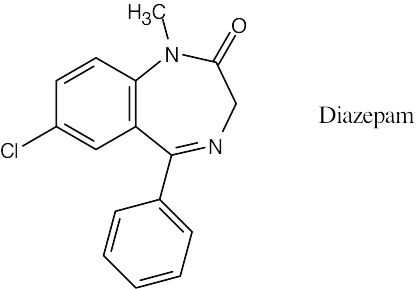
|
7.4 |
(1) |
Find the longest chain containing the double bond and name it. In (a), the longest chain is a pentene. |
|
(2) |
Identify the substituents. There are three methyl groups in (a). |
|
(3) |
Number the substituents, remembering that the double bond receives the lowest possible number. The methyl groups are attached to C3 and C4 (two methyl groups). |
|
(4) |
Name the compound, remembering to use the prefix “tri-” before “methyl” and remembering to use a number to signify the location of the double bond. The name of the compound in (a) is 3,4,4-trimethyl-1-pentene. |
|
(a) |
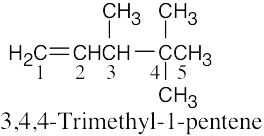 |
|
(b) |
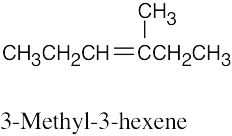 |
|
(c) |
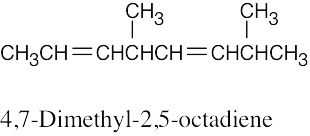 |
|
(d) |
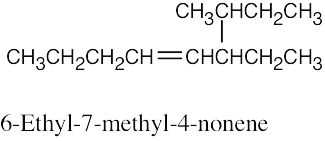 |
7.5 |
It is much easier to draw a structure from a given name than it is to name a structure. First, draw the carbon chain, placing the double bond or bonds in the designated locations. Then attach the cited groups in the proper positions. |
|
(a) |
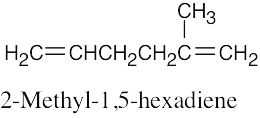 |
|
(b) |
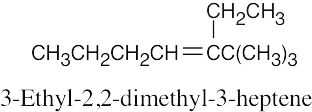 |
|
(c) |
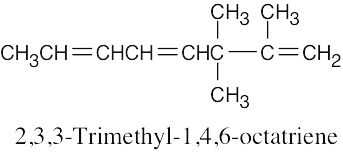 |
|
(d) |
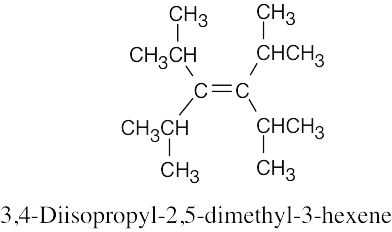 |
7.7 |
In the new naming system, the bond locant appears directly before –ene or –diene. |
|
(a) |
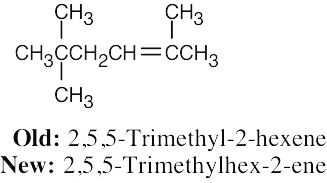 |
|
(b) |
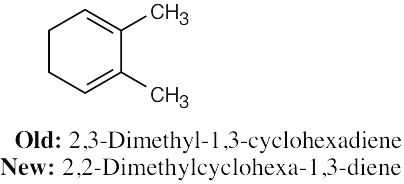 |
7.8 |
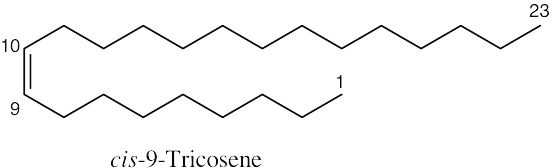 |
7.9 |
Compounds (c), (e), and (f) can exist as cis–trans isomers. |
|
(c) |
 |
|
(e) |
 |
|
(f) |
 |
7.10 |
(a) |
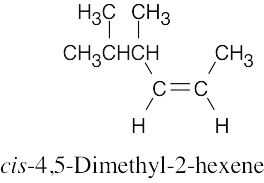 |
|
(b) |
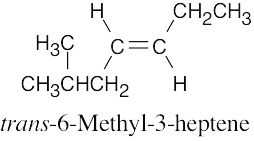 |
7.11 |
Review the sequence rules presented in Section 7.5. A summary: |
|
Rule 1: |
An atom with a higher atomic number has priority over an atom with a lower atomic number. |
|
Rule 2: |
If a decision can’t be reached by using Rule 1, look at the second, third, or fourth atom away from the double-bond carbon until a decision can be made. |
|
Rule 3: |
Multiple-bonded atoms are equivalent to the same number of single-bonded atoms. |
|
|
Higher |
Lower |
Rule |
(a) |
–CH3 |
–H |
1 |
(b) |
–Cl |
–CH2Cl |
1 |
(c) |
–CH=CH2 |
–CH2CH2Br |
3 |
(d) |
–OCH3 |
–NHCH3 |
1 |
(e) |
–CH=O |
–CH2OH |
3 |
(f) |
–CH=O |
–CH2OCH3 |
3 |
|
7.12 |
Highest priority ⎯⎯⎯→ Lowest Priority |
|
(a) |
–Cl, –OH, –CH3, –H |
|
(b) |
–CH2OH, –CH=CH2, –CH2CH3, –CH3 |
|
(c) |
–CO2H, –CH2OH, –C≡N, –CH2NH2 |
|
(d) |
–CH2OCH3, –C≡N, –C≡CH, –CH2CH3 |
7.14 |
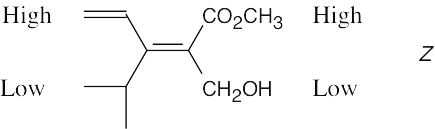 |
7.15 |
|
More stable Less stable |
|
(a) |
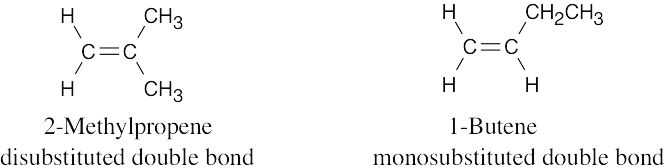 |
|
(b) |
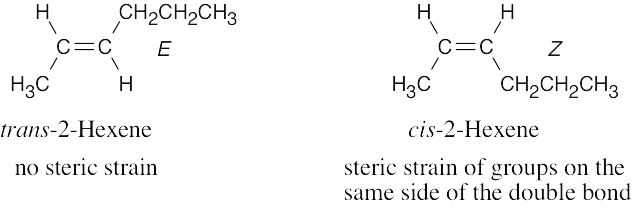 |
|
(c) |
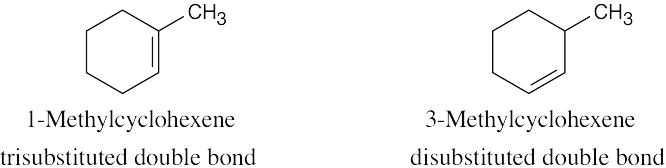 |
7.17 |
Think backward in choosing the alkene starting material for synthesis of the desired haloalkanes. Remember that halogen is bonded to one end of the double bond and that more than one starting material can give rise to the desired product. |
|
(a) |
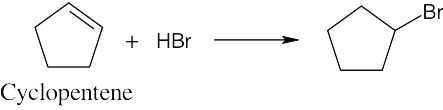 |
|
(b) |
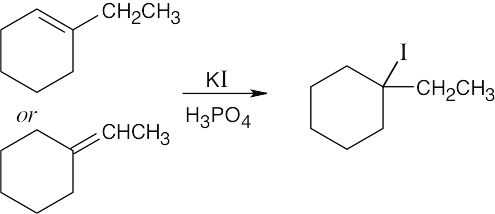 |
|
(c) |
 |
|
(d) |
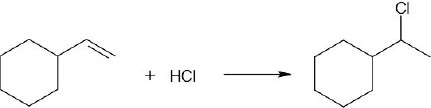 |
7.18 |
The more stable carbocation is formed. |
|
(a) |
 |
|
(b) |
 |
7.19 |
Two representations of the secondary carbocation are shown. In the alignment shown in the drawing, only one hydrogen (circled) is in the correct position for hyperconjugative overlap with the carbocation carbon.
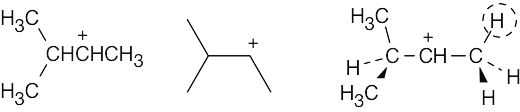
|
7.20 |
The second step in the electrophilic addition of HCl to an alkene is exergonic. According to the Hammond postulate, the transition state should resemble the carbocation intermediate.
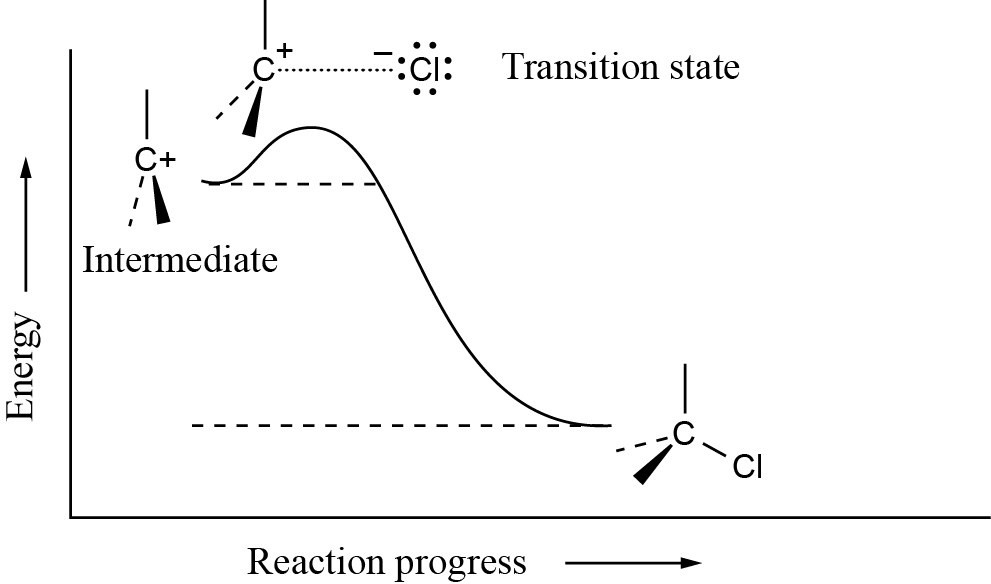
|
7.21 |
 |
|
Step 1: |
Electrophilic addition of H+ to double bond. |
|
Step 2: |
Hydride shift that forms a more stable tertiary carbocation. |
|
Step 3: |
Reaction of carbocation with Br–. |
Additional Problems
Visualizing Chemistry
7.25 |
 |
Mechanism Problems
7.26 |
(a) |
Mechanism:

|
|
(b) |
Mechanism:

|
|
(c) |
Mechanism:

|
7.27 |
(a) |
Mechanism:

|
|
(b) |
Mechanism:

|
|
(c) |
Mechanism:
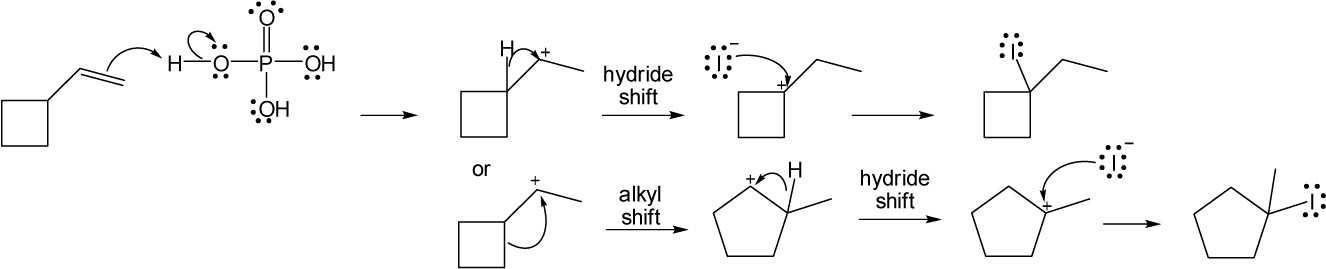
|
7.28 |
Note: Because the carbocation intermediate is resonance stabilized, there are two locations where the bromine can add.

Mechanism:

|
7.30 |
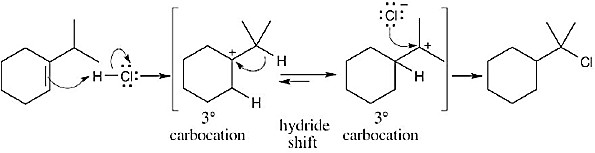 |
7.31 |
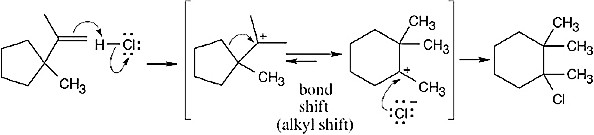 |
7.32 |
Step 2, in which the double bond electrons add to the carbocation, is an alkene electrophilic addition.
|
7.33 |
Steps 1 and 2 are alkene electrophilic additions, and steps 3 and 4 involve carbocation rearrangements..
|
Calculating a Degree of Unsaturation
7.34 |
The purpose of this problem is to give you experience in calculating the number of double bonds and/or rings in a formula. Additionally, you will learn to draw structures containing various functional groups. Remember that any formula that satisfies the rules of valency is acceptable. Try to identify functional groups in the structures that you draw. Many structures are acceptable for each part of this problem. |
|
(a) |
C10H16 – 3 degrees of unsaturation. Examples:
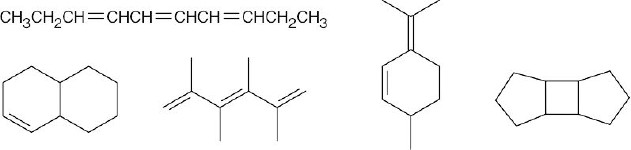
|
|
(b) |
C8H8O. The equivalent hydrocarbon is C8H8, which has 5 degrees of unsaturation.
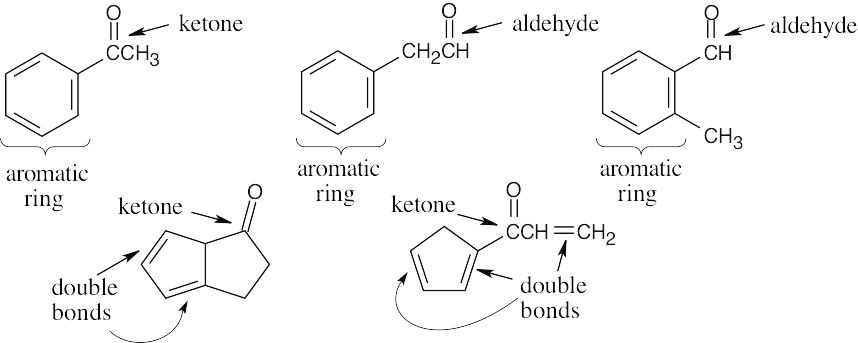
|
|
(c) |
C7H10Cl2 has C7H12 as its equivalent hydrocarbon formula. C7H10Cl2 has two degrees of unsaturation.
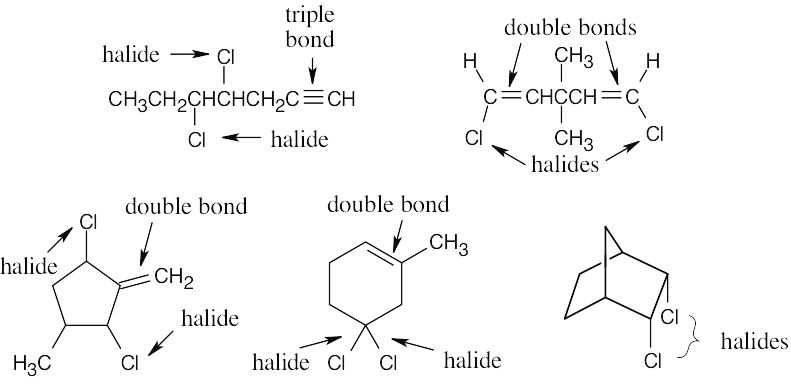
|
|
(d) |
C10H16O2 – 3 degrees of unsaturation (C10H16 = equivalent hydrocarbon formula).
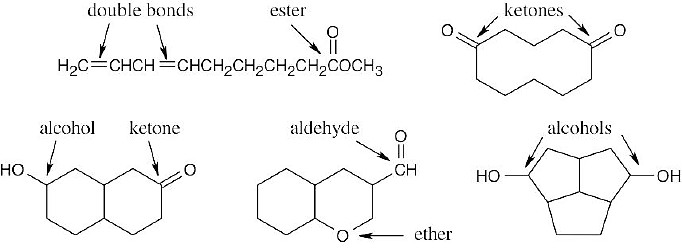
|
|
(e) |
C5H9NO2 – 2 degrees of unsaturation (C5H8 = equivalent hydrocarbon formula).
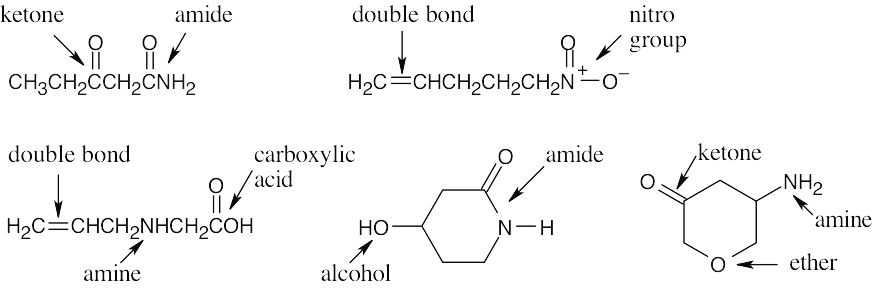
|
|
(f) |
C8H10ClNO – 4 degrees of unsaturation (C8H10 = equivalent hydrocarbon formula).
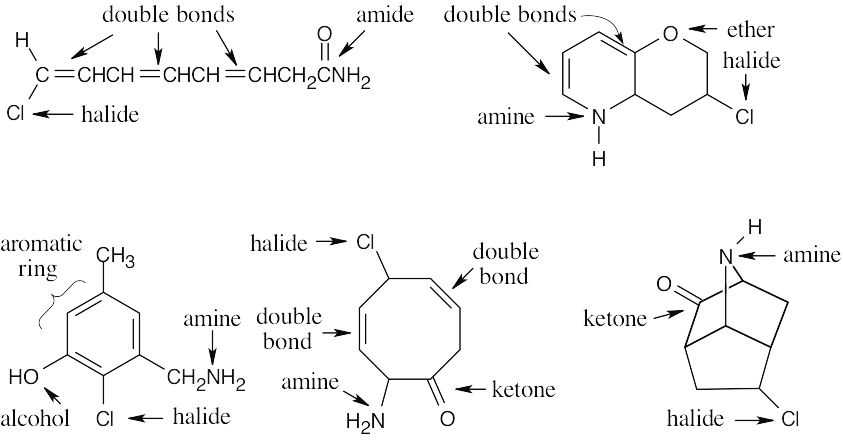
|
7.35 |
Compound |
Equivalent hydrocarbon formula |
Degree of unsaturation |
Complete formula |
(a) |
C8H?O2 |
C8H18 |
3 |
C8H12O2 |
(b) |
C7H?N |
C7H16 |
2 |
C7H13N |
(c) |
C9H?NO |
C9H20 |
4 |
C9H13NO |
7.36 |
Solve this problem in the same way as we solved problems 7.3 and 7.27. A C22 hydrocarbon with 12 degrees of unsaturation (four rings and eight double bonds) has a formula C22H46 – H24 = C22H22. Adding two hydrogens (because of the two nitrogens) and subtracting one hydrogen (because of the chlorine), gives the formula C22H23ClN2O2 for Loratadine.
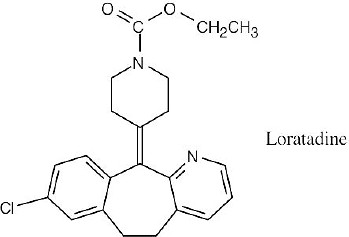
|
Naming Alkenes
7.37 |
(a) |
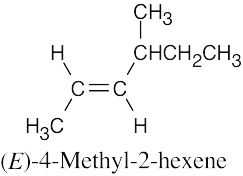 |
|
(b) |
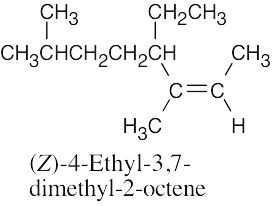 |
|
(c) |
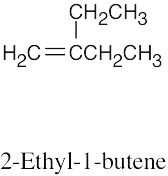 |
|
(d) |
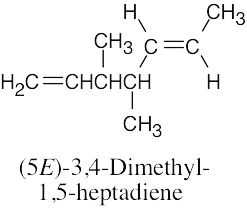 |
|
(e) |
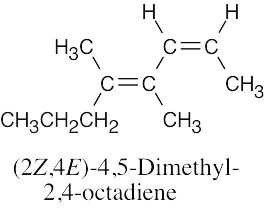 |
|
(f) |
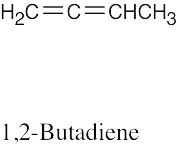 |
7.38 |
(a) |
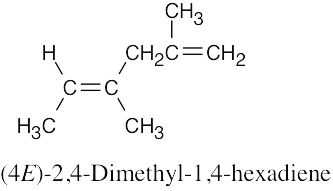 |
|
(b) |
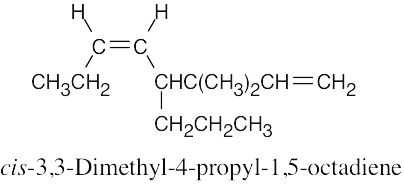 |
|
(c) |
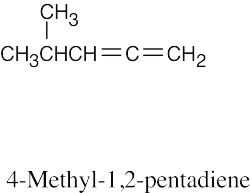 |
|
(d) |
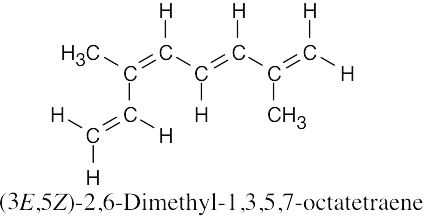 |
|
(e) |
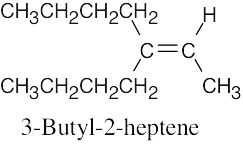 |
|
(f) |
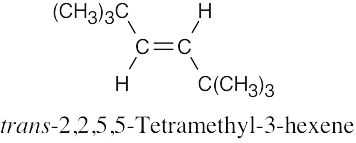 |
7.39 |
(a) |
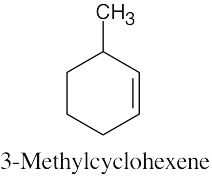 |
|
(b) |
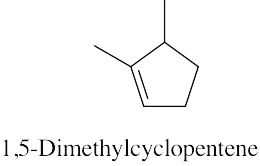 |
|
(c) |
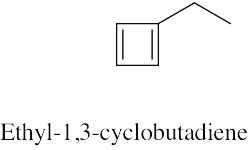 |
|
(d) |
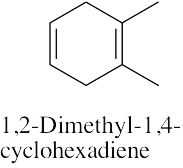 |
|
(e) |
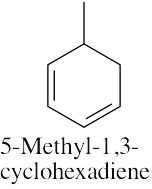 |
|
(f) |
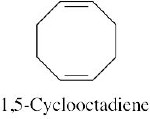 |
7.40 |
Because the longest carbon chain contains 8 carbons and 3 double bonds, ocimene is an octatriene. Start numbering at the end that will give the lower number to the first double bond (1,3,6 is lower than 2,5,7). Number the methyl substituents and, finally, name the compound.

|
7.41 |
(3E,6E)-3,7,11-Trimethyl-1,3,6,10-dodecatetraene
|
7.42 |
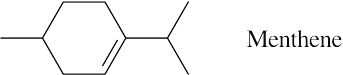 |
7.43 |
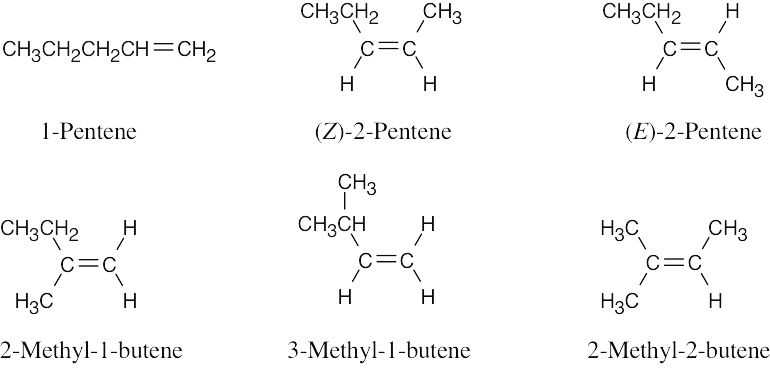 |
7.44 |
Start with 1-hexene and continue on until all hexenes are named, making sure that E,Z designations have been made when necessary. Then move on to all 1-pentenes, 2- pentenes, etc.
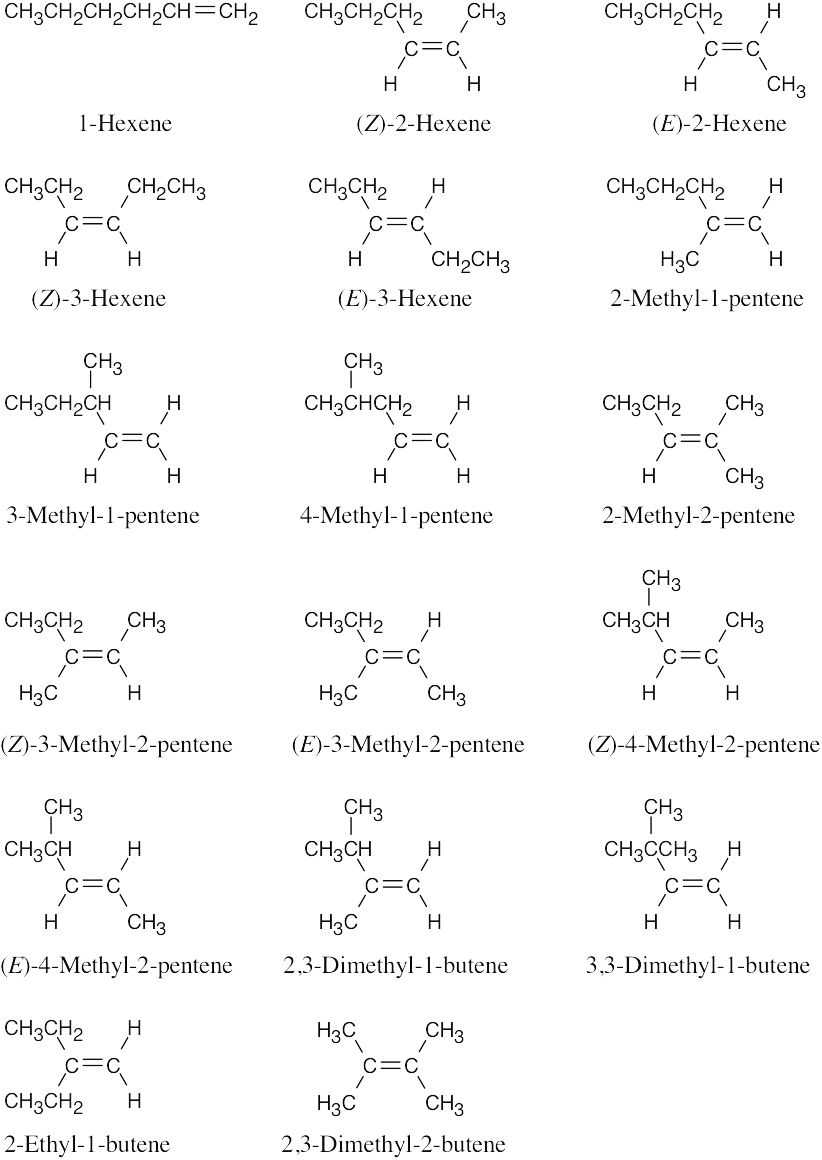
|
Alkene Isomers and Their Stability
7.45 |
Highest priority ⎯⎯⎯→ Lowest Priority |
|
(a) |
–I, –Br, –CH3, –H |
|
(b) |
–OCH3, –OH, –CO2H, –H |
|
(c) |
–CO2CH3, –CO2H, –CH2OH, –CH3 |
|
(d) |
–COCH3, –CH2CH2OH, –CH2CH3, –CH3 |
|
(e) |
–CH2Br, –C≡N, –CH2NH2, –CH=CH2 |
|
(f) |
–CH2OCH3, –CH2OH, –CH=CH2, CH2CH3 |
7.47 |
(a) |
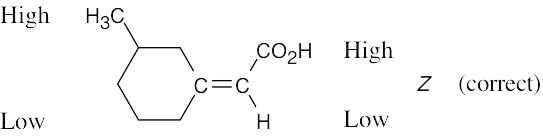 |
|
(b) |
 |
|
(c) |
 |
|
(d) |
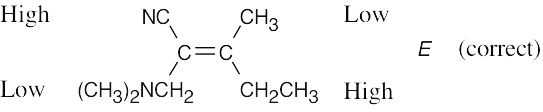 |
|
(e) |
 |
|
(f) |
 |
7.49 |
As expected, the two trans compounds are more stable than their cis counterparts. The cis– trans difference is much more pronounced for the tetramethyl compound, however. Build a model of cis-2,2,5,5-tetramethyl-3-hexene and notice the extreme crowding of the methyl groups. Steric strain makes the cis isomer much less stable than the trans isomer and causes cis ΔH°hydrog to have a much larger negative value than trans ΔH°hydrog for the hexene isomers.
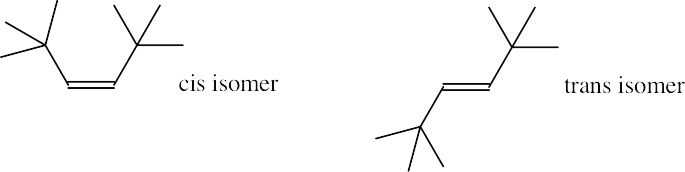
|
7.50 |
A model of cyclohexene shows that a six-membered ring is too small to contain a trans double bond without causing severe strain to the ring. A ten-membered ring is flexible enough to accommodate either a cis or a trans double bond, although the cis isomer has less strain than the trans isomer. |
7.51 |
Build models of the two cyclooctenes and notice the large amount of torsional strain in trans-cyclooctene relative to cis-cyclooctene. This torsional strain, in addition to angle strain, causes the trans isomer to be of higher energy and to have a ΔH°hydrog larger than the ΔH°hydrog of the cis isomer. |
7.52 |
Models show that the difference in strain between the two cyclononene isomers is smaller than the difference between the two cyclooctene isomers. This reduced strain is due to a combination of less angle strain and more puckering to relieve torsional strain and is reflected in the fact that the values of ΔH°hydrog for the two cyclononene isomers are relatively close. Nevertheless, the trans isomer is still more strained than the cis isomer. |
7.53 |
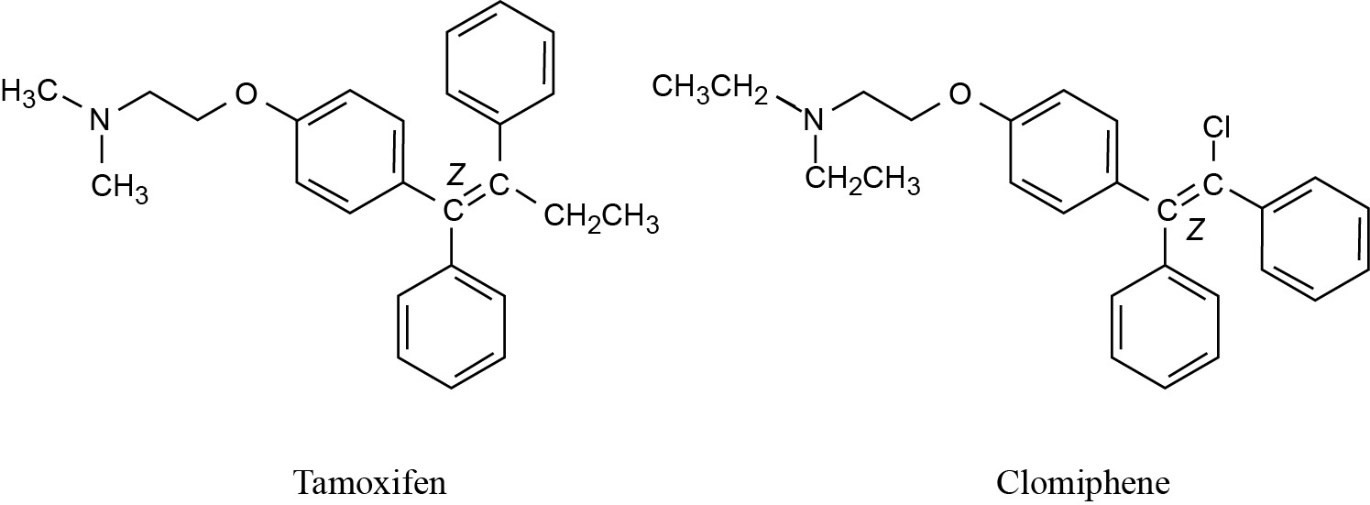
|
Carbocations and Electrophilic Addition Reactions
7.56 |
Carbocations can be resonance stabilized. Draw all the resonance forms that would stabilize each carbocation. |
|
(a) |
 |
|
(b) |
 |
|
(c) |
 |
7.58 |
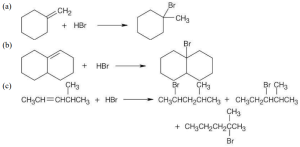 |
|
|
|
The third product results from rearrangement to a more stable tertiary carbocation. |
7.60 |
(a) |
The primary carbocation rearranges to the more stable secondary carbocation by a hydride shift.
|
|
(b) |
This hydride shift produces a tertiary carbocation from rearrangement of a secondary carbocation.
|
|
(c) |
An alkyl shift forms a tertiary carbocation from a primary carbocation. In this example, rearrangement involves migration of the electrons from one of the cyclobutane ring bonds to form a cyclopentane ring.
|
General Problems
7.61 |
The central carbon of allene forms two σ bonds and two π bonds. The central carbon is sp– hybridized, and the carbon–carbon bond angle is 180°, indicating linear geometry for the carbons of allene. The hydrogen atoms on one terminal =CH2 units are perpendicular to the pair of hydrogen atoms on the other =CH2 group.
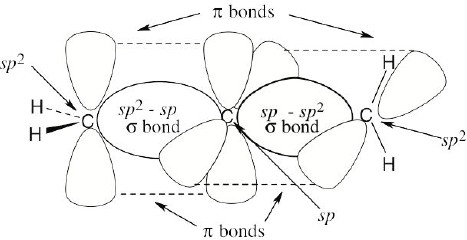
|
7.62 |
The heat of hydrogenation for a typical diene is 2 × (ΔH°hydrog of an alkene) = –252 kJ/mol. Thus, allene, with ΔH°hydrog = –295 kJ/mol is 43 kJ/mol higher in energy than a typical diene and is less stable. |
7.63 |
Retin A contains five carbon–carbon double bonds. Since the ring double bond cannot isomerize, Retin A can have 24 = 16 isomers.
|
7.64 |
(a) |
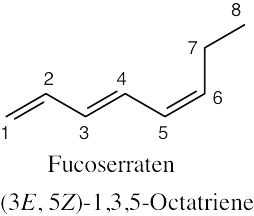 |
|
(b) |
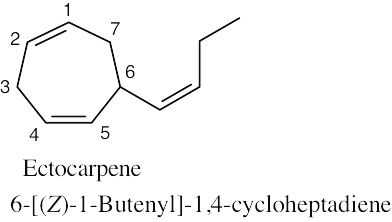 |
7.65 |
Treatment of the tert-butyl ester with trifluoroacetic acid cleaves the –OC(CH3)3 group and replaces it with an –OH group, which has a lower priority than the –OCH3 group on the upper carbon and the –OC(CH3)3 group that was removed. The result is a change in the E,Z designation around the double bond without breaking any of the bonds attached to the double-bond carbons.
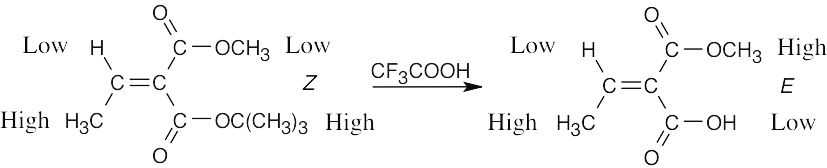
|
7.66 |
Attack of the π electrons of the double bond on H+ yields the carbocation pictured on the far right. A bond shift (alkyl shift) produces the bracketed intermediate, which reacts with Br– to yield 1-bromo-2-methylcyclobutane.
|
7.67 |
(a) |
C27H46O |
5 degrees of unsaturation |
|
(b) |
C14H9Cl6 |
8 degrees of unsaturation |
|
(c) |
C20H34O5 |
4 degrees of unsaturation |
|
(d) |
C8H10N4O2 |
6 degrees of unsaturation |
|
(e) |
C21H28O5 |
8 degrees of unsaturation |
|
(f) |
C17H23NO3 |
7 degrees of unsaturation |
7.68 |
The reaction is exergonic because it is spontaneous. According to the Hammond postulate, the transition state should resemble the isobutyl cation.
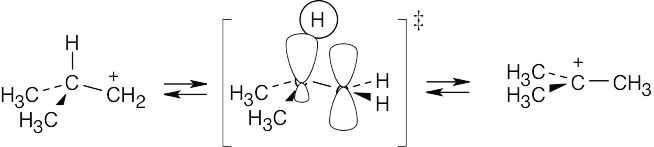
|
7.69 |
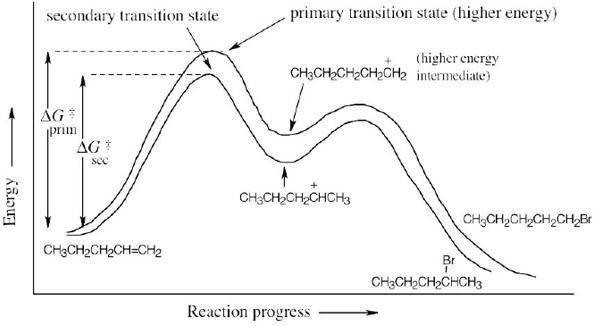 |
7.70 |
|
Transition State #1 |
Transition State #2
|
|
2-Bromopentane path |
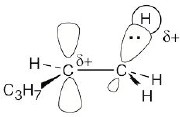 |
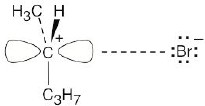 |
|
1-Bromopentane path |
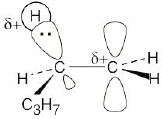 |
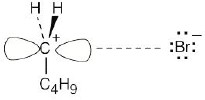 |
|
The first step (carbocation formation) is endergonic for both reaction paths, and both transition states resemble the carbocation intermediates. Transition states for the exergonic second step also resemble the carbocation intermediate. |
7.71 |
Reaction of 1-chloropropane with the Lewis acid AlCl3 forms a carbocation. The less stable propyl carbocation (primary), undergoes a hydride shift to produce the more stable isopropyl carbocation (secondary), which reacts with benzene to give isopropylbenzene.
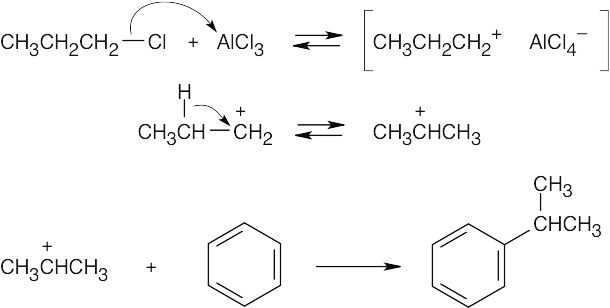
|
7.72 |
The product, 2,3-dimethyl-2-butene, is formed by elimination of HBr from 2-bromo-2,3- dimethylbutane. This product forms because it has the more substituted double bond.
|
This file is copyright 2023, Rice University. All Rights Reserved.