21 Chapter 21 – Carboxylic Acid Derivatives: Nucleophilic Acyl Substitution Reactions
21.1 |
Table 21.1 lists the suffixes for naming carboxylic acid derivatives. The suffixes used when the functional group is part of a ring are in parentheses. |
|
(a) |
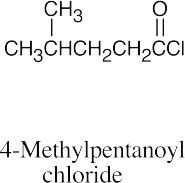 |
(b) |
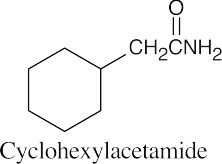 |
|
(c) |
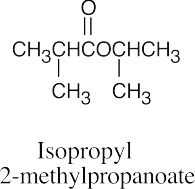 |
(d) |
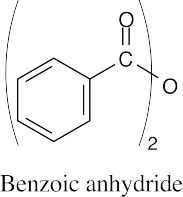 |
|
(e) |
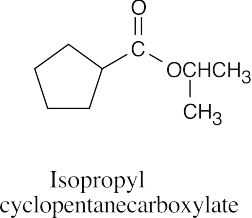 |
(f) |
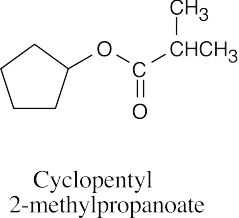 |
|
(g) |
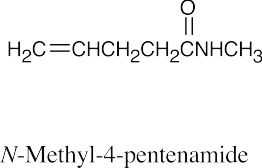 |
(h) |
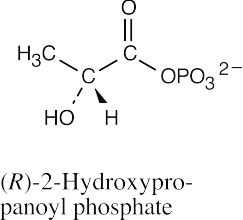 |
|
(i) |
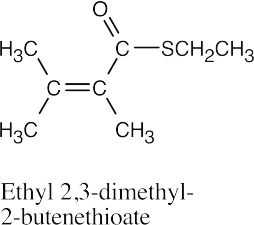 |
|
|
21.2 |
(a) |
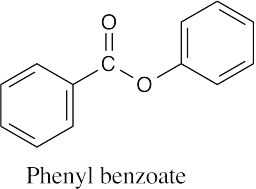 |
(b) |
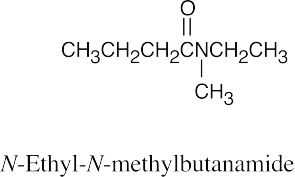 |
|
(c) |
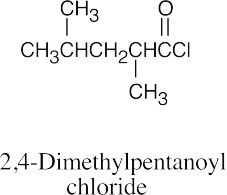 |
(d) |
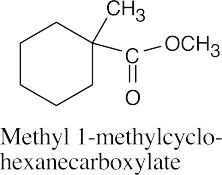 |
|
(e) |
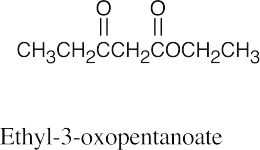 |
(f) |
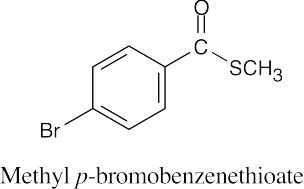 |
|
(g) |
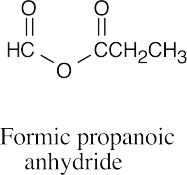 |
(h) |
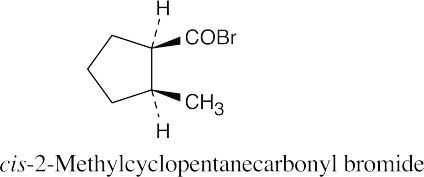 |
21.3 |
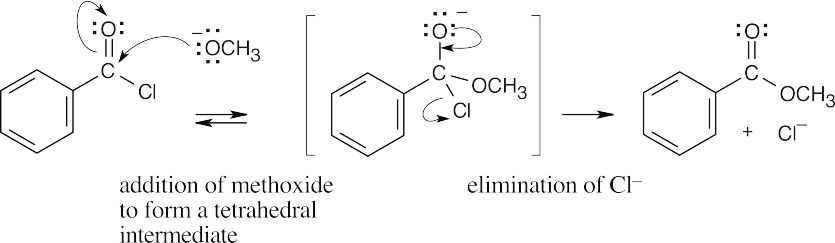 |
21.8 |
Under Fischer esterification conditions, many hydroxycarboxylic acids can form intramolecular esters (lactones).
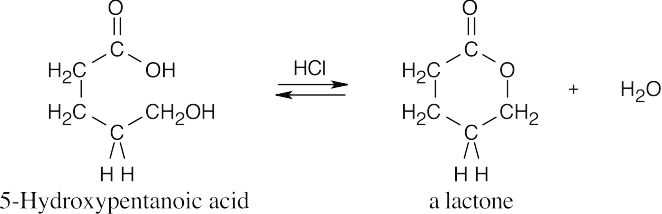
|
21.11 |
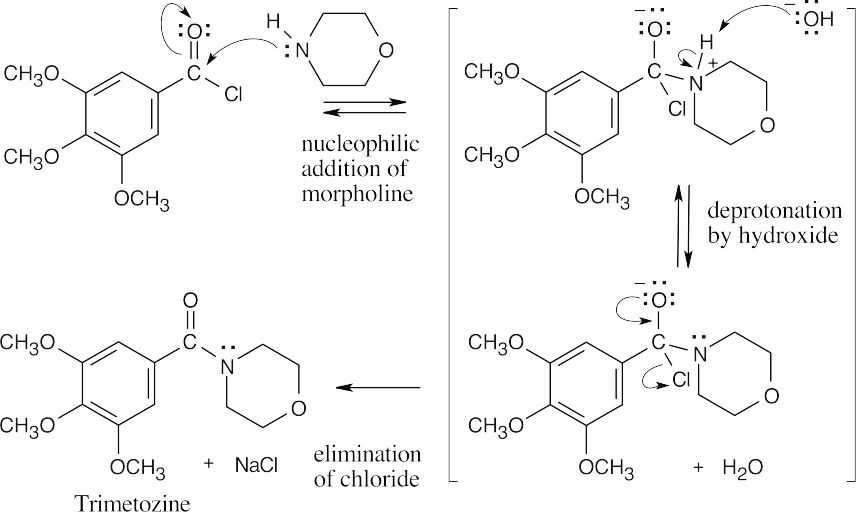 |
21.12 |
An extra equivalent of base must be added to neutralize the acid produced in these reactions. In (a) and (b), two equivalents of the amine may be used in place of NaOH. |
|
(a) |
 |
|
(b) |
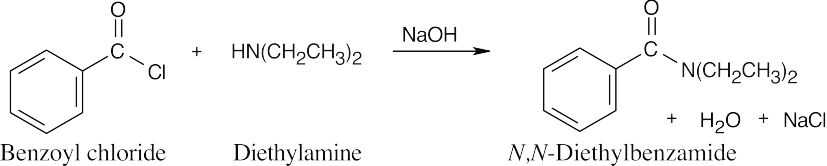 |
|
(c) |
 |
21.13 |
Two combinations of acid chloride and organocopper reagent are possible. |
|
(a) |
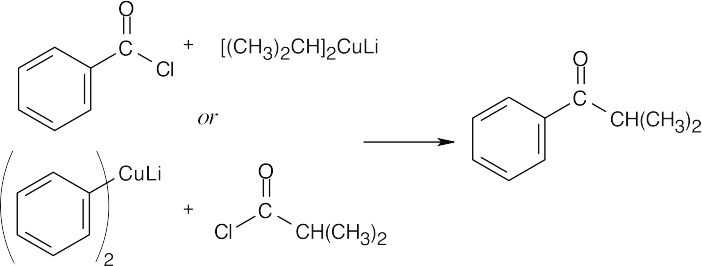 |
|
(b) |
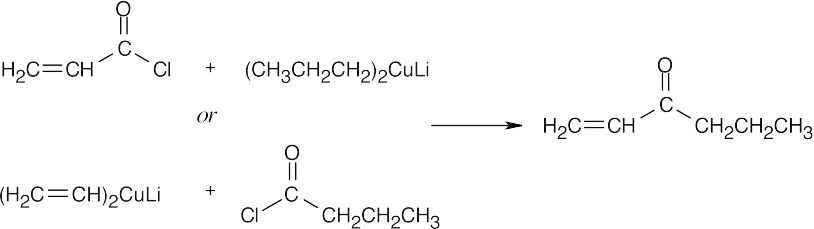 |
21.14 |
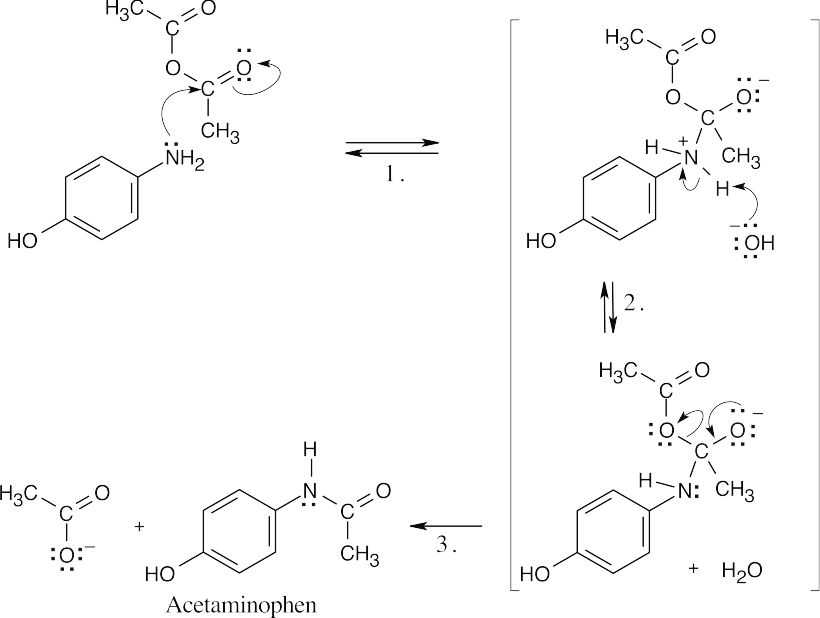 |
|
Step 1: |
Nucleophilic addition of p-hydroxyaniline. |
|
Step 2: |
Deprotonation by hydroxide. |
|
Step 3: |
Loss of acetate ion. |
21.15 |
The second half of the anhydride becomes a carboxylic acid.
|
21.16 |
Acidic hydrolysis of an ester is a reversible reaction because the products are an alcohol and a carboxylic acid. Basic hydrolysis of an ester is irreversible because its products are an alcohol and a carboxylate anion, which has a negative charge and does not react with nucleophiles. |
21.17 |
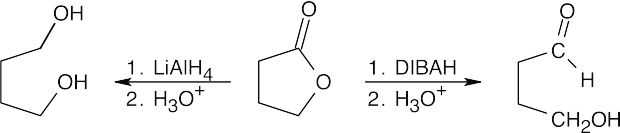 |
21.18 |
Lithium aluminum hydride reduces an ester to form two alcohols. |
|
(a) |
 |
|
(b) |
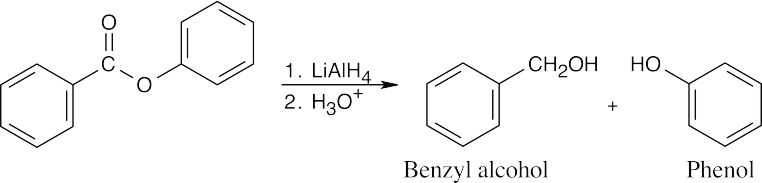 |
21.19 |
Remember that Grignard reagents can only be used with esters to form a tertiary alcohol that has two identical substituents. Identify these two substituents, which come from the Grignard reagent, and work backward to select the ester (the alkyl group of the ester is unimportant).
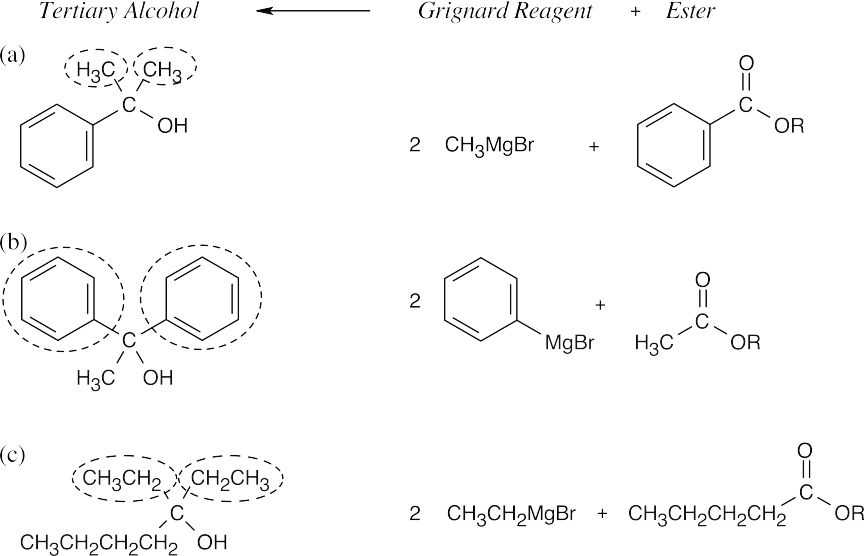
|
21.20 |
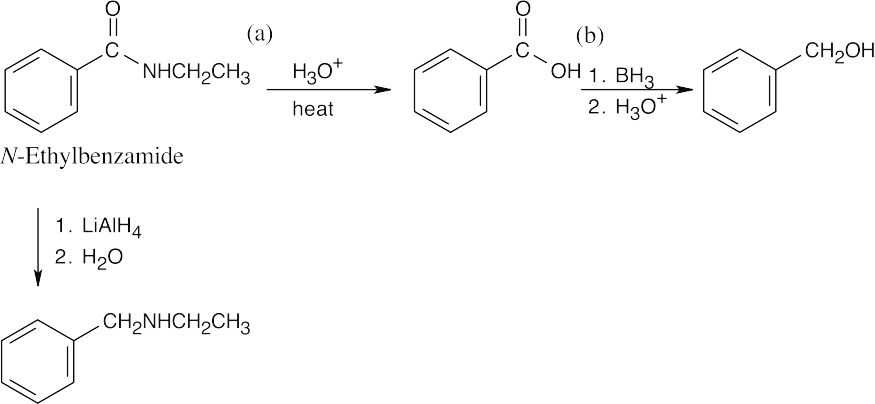 |
21.21 |
The product is an N,N-disubstituted amine, which can be formed by reduction of an amide. The amide results from treatment of an acid chloride with the appropriate amine. The acid chloride is the product of the reaction of SOCl2 with a carboxylic acid that is formed by carboxylation of the Grignard reagent synthesized from the starting material.
|
21.22 |
Even though the entire molecule of coenzyme A is biologically important, we are concerned in this problem only with the –SH group. The remainder of the structure is represented here as “R”.
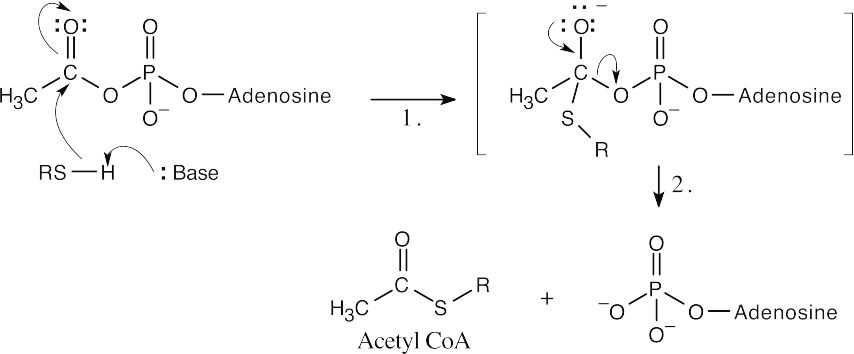
|
|
Step 1: |
Nucleophilic addition of the –SR group of CoA (after deprotonation) to acetyl adenylate to form a tetrahedral intermediate. |
|
Step 2: |
Loss of adenosine monophosphate. |
21.23 |
In each example, if n molecules of one component react with n molecules of the other component, a polymer with n repeating units is formed, and 2n small molecules are formed as byproducts; these are shown in each reaction. |
|
(a) |
 |
|
(b) |
 |
|
(c) |
 |
21.24 |
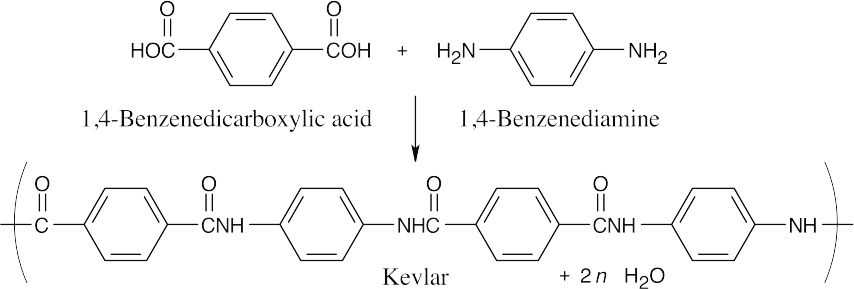 |
21.25 |
Use Table 21.3 if you need help. |
|
Absorption: |
Functional group present: |
|
(a) |
1735 cm–1 |
Saturated ester or 6-membered ring lactone |
|
(b) |
1810 cm–1 |
Saturated acid chloride |
|
(c) |
2500 – 3300 cm–1 and 1710 cm–1 |
Carboxylic acid |
|
(d) |
1715 cm–1 |
Saturated ketone or 6-membered ring ketone |
21.26 |
(a) |
IR 1735 cm–1 corresponds to a saturated ester.
The remaining five carbons and twelve hydrogens can be arranged in a number of ways to produce a structure for this compound. For example:

The structural formula indicates that this compound can’t be a lactone.
|
|
(b) |
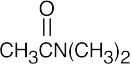 |
|
(c) |
 |
Additional Problems
Visualizing Chemistry
21.27 |
(a) |
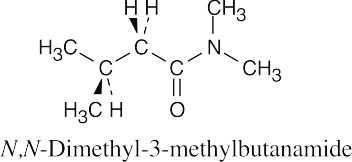 |
(b) |
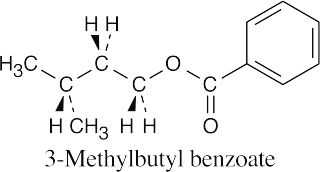 |
|
|
21.28 |
(a) |
This compound can also be synthesized by Fischer esterification of o-bromobenzoic acid with 2-propanol and an acid catalyst.
|
|
(b) |
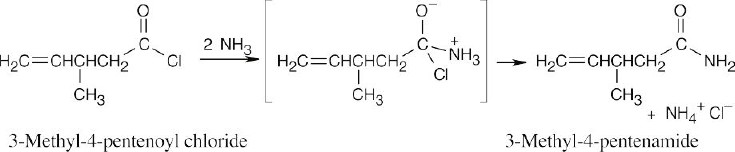
|
21.29 |
The starting material is 3-methyl-4-pentenoyl chloride, as indicated by the –Cl in the tetrahedral intermediate. Ammonia adds to give the observed tetrahedral intermediate, which eliminates Cl– to yield the above amide. |
21.30 |
According to the electrostatic potential maps, the carbonyl carbon of acetyl azide is more electron-poor (less red) and therefore more reactive in nucleophilic acyl substitution reactions. Resonance donation of nitrogen lone-pair electrons to the carbonyl group is greater in an amide than in an acyl azide.
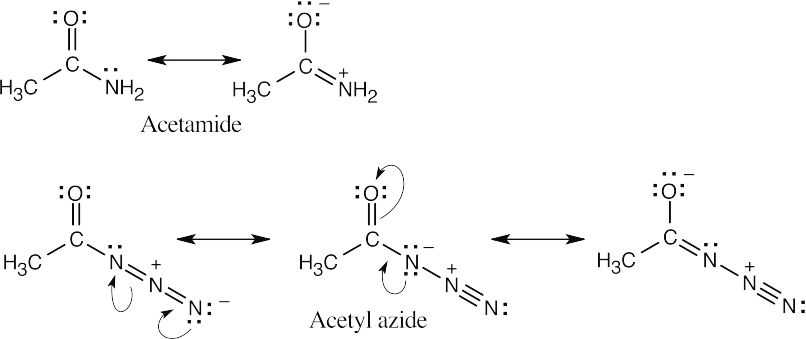
|
Mechanism Problems
21.31 |
(a) |
Mechanism:

|
|
(b) |
Mechanism:
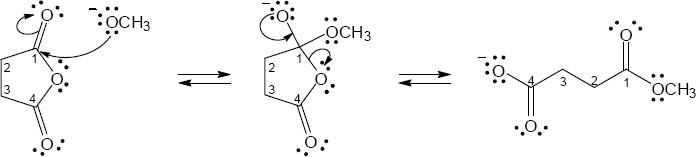
|
21.32 |
(a) |
Mechanism:
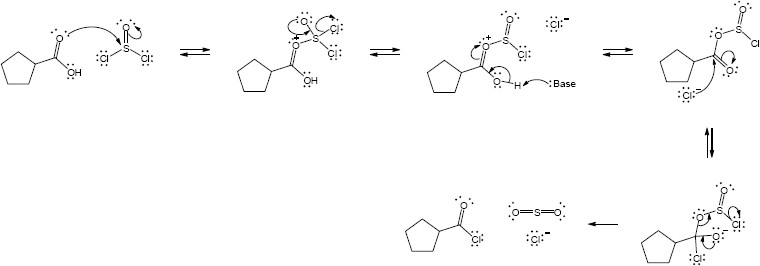
|
|
(b) |
Mechanism:
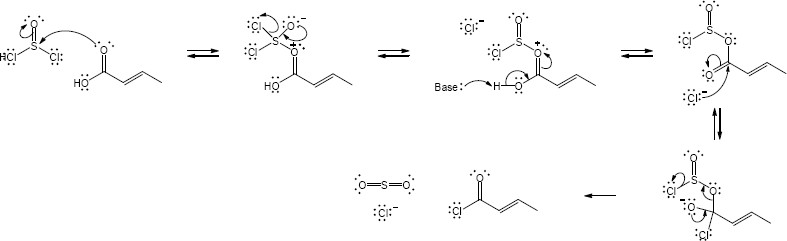
|
21.33 |
(a) |
Mechanism:
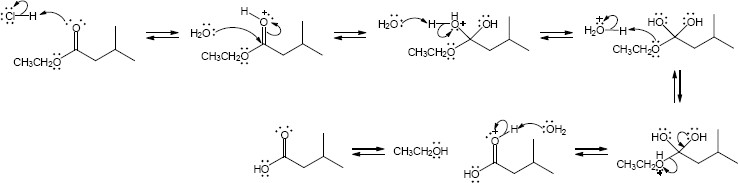
|
|
(b) |
Mechanism:
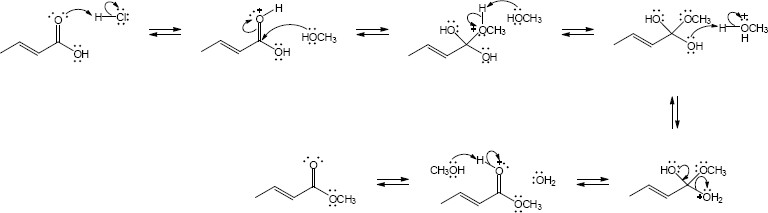
|
21.34 |
(a) |
Mechanism:
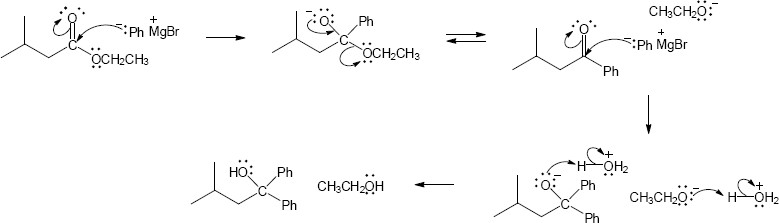
|
|
(b) |
Mechanism:
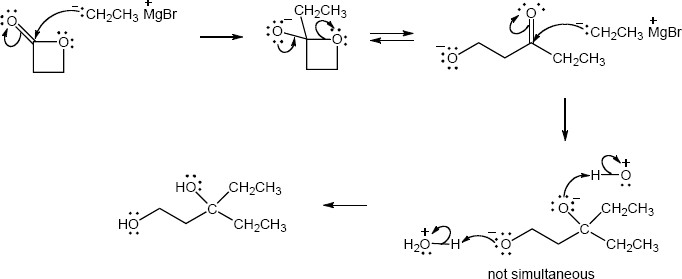
|
21.35 |
Note: Two factors are responsible for the observed regioselectivity. First, the sterics of the tert-butyl group block the nucleophile from reacting at the adjacent carbonyl. Second, the nitrogen of the amino acid has an inductive effect increasing the electrophilic character of the amino acid carbonyl.

Mechanism:

|
21.36 |
Mechanism:
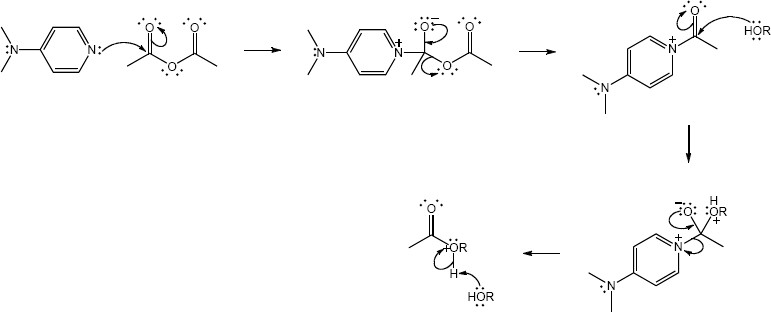
|
21.37 |
Addition of –OH to the fatty acyl CoA (Step 1), followed by loss of –SCoA from the tetrahedral intermediate (Step 2), produces 1-acylglycerol 3-phosphate.
|
21.38 |
Formation of the dipeptide:
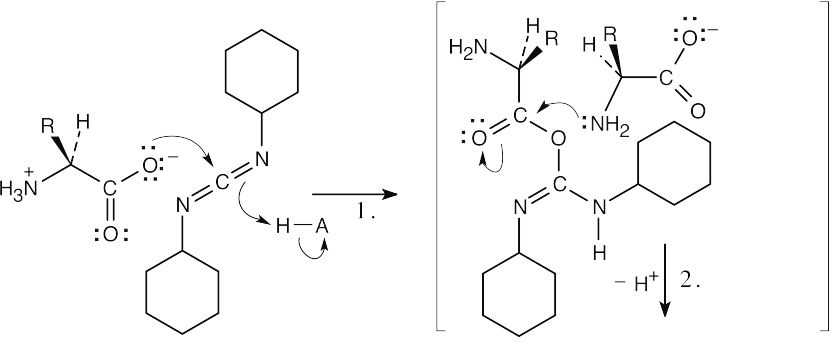
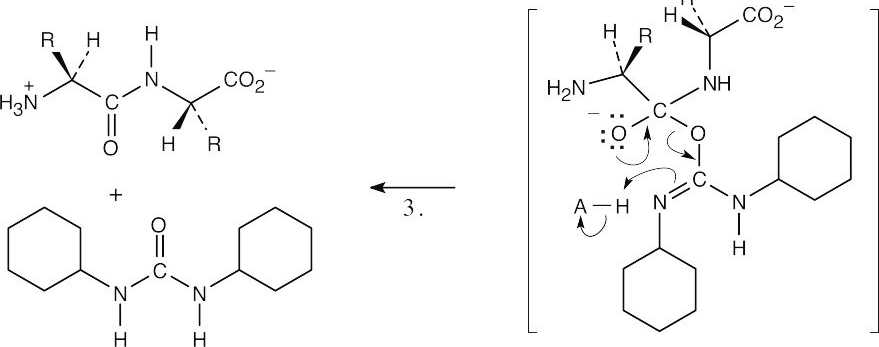
|
|
Step 1: |
The carboxylate group from one amino acid adds to DCC to form a reactive intermediate. |
|
Step 2: |
The amino group of the second amino acid adds to the carbonyl group to yield a tetrahedral intermediate. |
|
Step 3: |
The intermediate loses dicyclohexylurea to produce the amide. Proton transfers occur in steps 1 and 3. |
|
Formation of the 2,5-diketopiperazine:
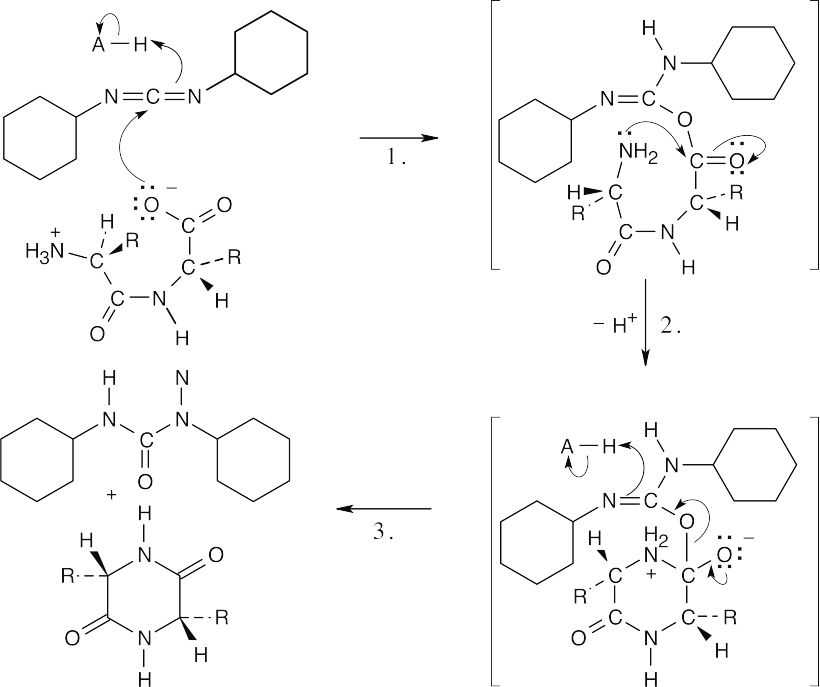
|
|
Step 1: |
Addition of carboxylate to DCC. |
|
Step 2: |
Intramolecular nucleophilic attack of the amino terminal end of the amide on the acylating agent. |
|
Step 3: |
Loss of dicyclohexylurea. Proton transfers occur in steps 1 and 2. |
|
Proton transfers occur in steps 1 and 2. |
21.39 |
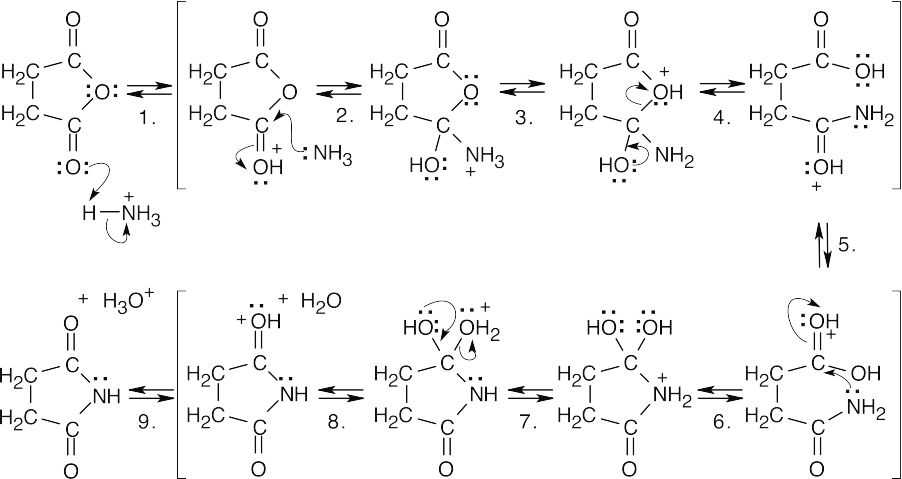 |
|
A summary of steps: |
|
Step 1: |
Protonation. |
|
Step 2: |
Nucleophilic addition of NH3. |
|
Step 3, 5, 7, 9: |
Proton transfers. |
|
Step 4: |
Ring opening. |
|
Step 6: |
Nucleophilic addition of –NH2. |
|
Step 8: |
Loss of H2O. |
|
This reaction requires high temperatures because the intermediate amide is a poor nucleophile and the carboxylic acid carbonyl group is unreactive. |
21.40 |
Both steps involve nucleophilic acyl substitutions.
Formation of acyl phosphate:
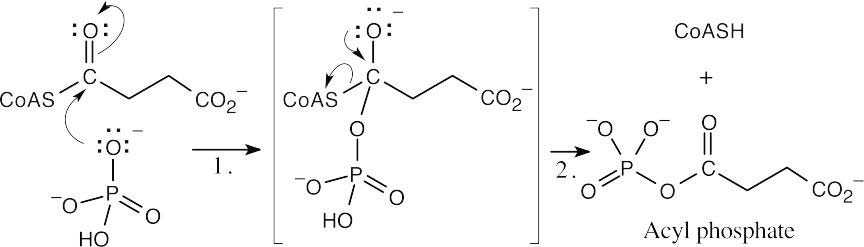
|
|
Step 1: |
Reaction of the phosphate oxygen with the carbonyl carbon of succinyl CoA. |
|
Step 2: |
Loss of –SCoA from the tetrahedral intermediate, yielding acyl phosphate. |
|
Conversion of acyl phosphate to succinate:
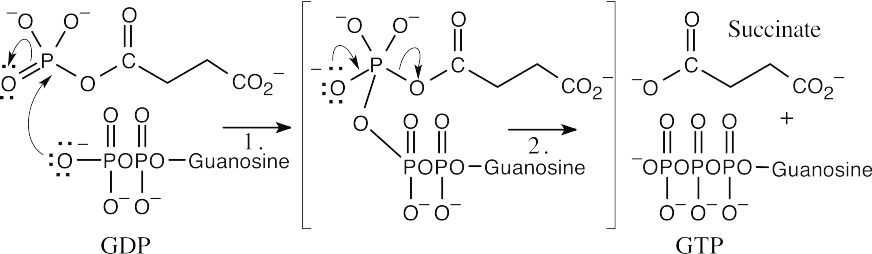
|
|
Step 1: |
Reaction of the diphosphate oxygen of GDP with the phosphorus of the acyl phosphate to produce an intermediate similar to the intermediates formed in nucleophilic acyl substitutions of carboxylic acid derivatives. |
|
Step 2: |
Loss of phosphate to form GTP and succinate. |
21.41 |
In all of these reactions, a nucleophile adds to either carbon or phosphorus to form an intermediate that expels a leaving group to give the desired product. |
Formation of 1,3-bisphosphoglycerate:
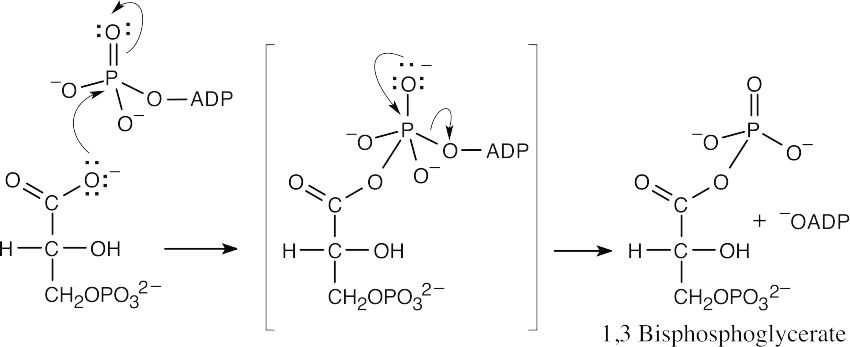
|
Route to enzyme-bound thioester:
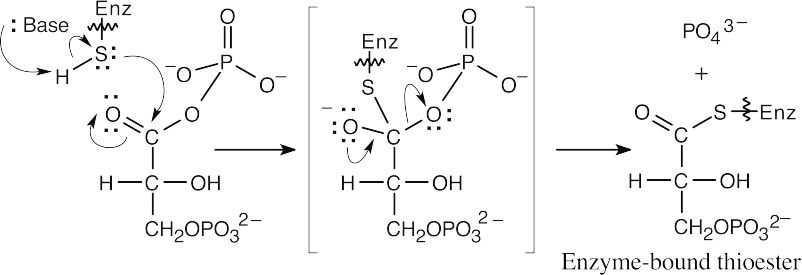
|
Reduction to glyceraldehyde 3-phosphate:
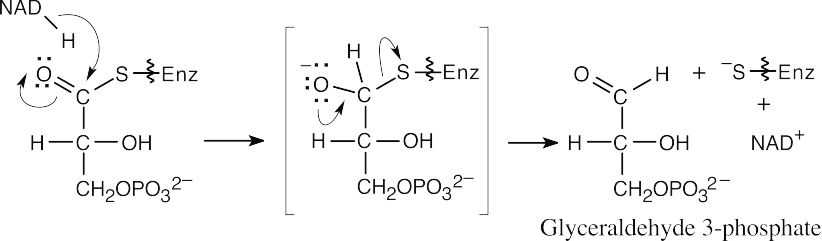
|
21.42 |
In (c), the imine rearranges to an α,β-unsaturated ester, to which the nucleophile adds to give the trapped β-lactamate.
|
21.43 |
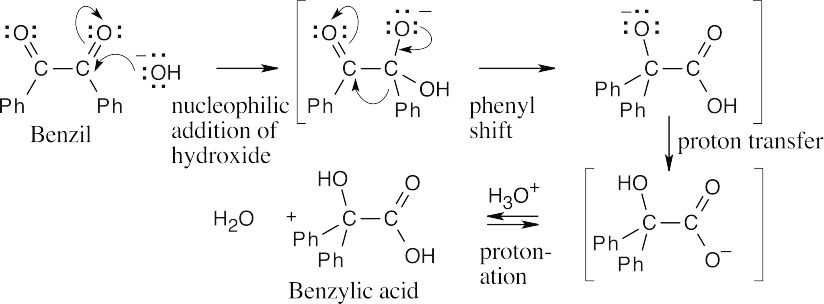 |
21.44 |
This is a nucleophilic acyl substitution reaction whose mechanism is similar to others we have studied.

I3C:– can act as a leaving group because the electron-withdrawing iodine atoms stabilize the carbanion.
|
Naming Carboxylic Acid Derivatives
21.45 |
(a) |
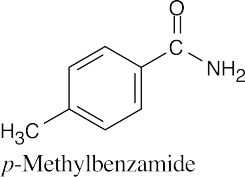 |
(b) |
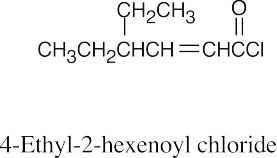 |
|
(c) |
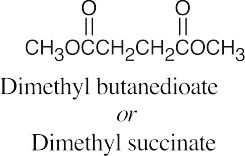 |
(d) |
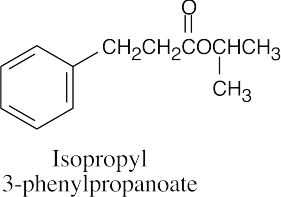 |
|
(e) |
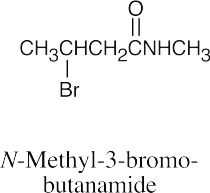 |
(f) |
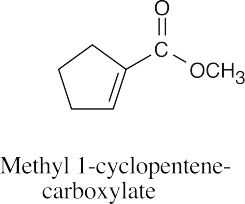 |
|
(g) |
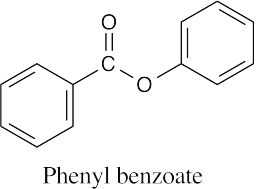 |
(h) |
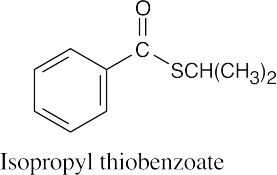 |
21.46 |
(a) |
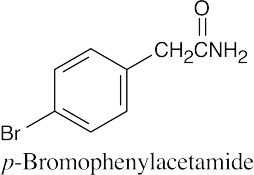 |
(b) |
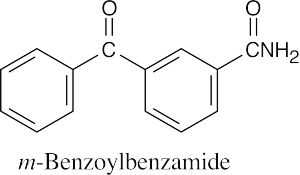 |
|
(c) |
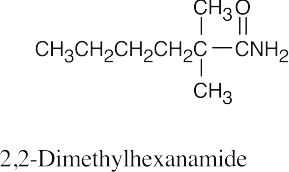 |
(d) |
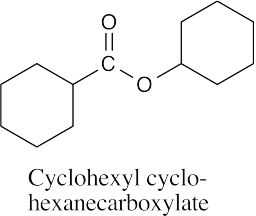 |
|
(e) |
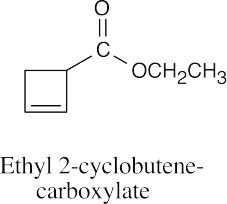 |
(f) |
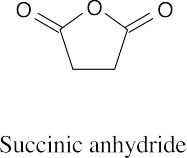 |
21.47 |
Many structures can be drawn for each part of this problem. |
|
(a) |
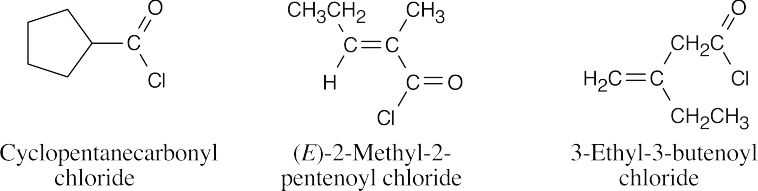 |
|
(b) |
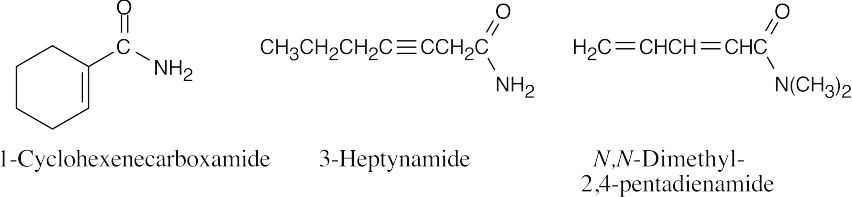 |
Nucleophilic Acyl Substitution Reactions
21.48 |
(a) |
 |
|
(b) |
 |
|
(c) |
 |
|
(d) |
 |
|
(e) |
 |
|
(f) |
 |
|
(g) |
 |
21.49 |
The reagents in parts (a), (e), and (g) don’t react with methyl propanoate. |
|
(b) |
 |
|
(c) |
 |
|
(d) |
 |
|
(f) |
 |
21.50 |
The reagents in parts (a), (e), (f), and (g) don’t react with propanamide. |
|
(b)
(c)
(d) |
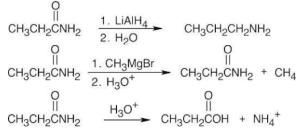 |
21.51 |
Dimethyl carbonate is a diester. Use your knowledge of the Grignard reaction to work your way through this problem.
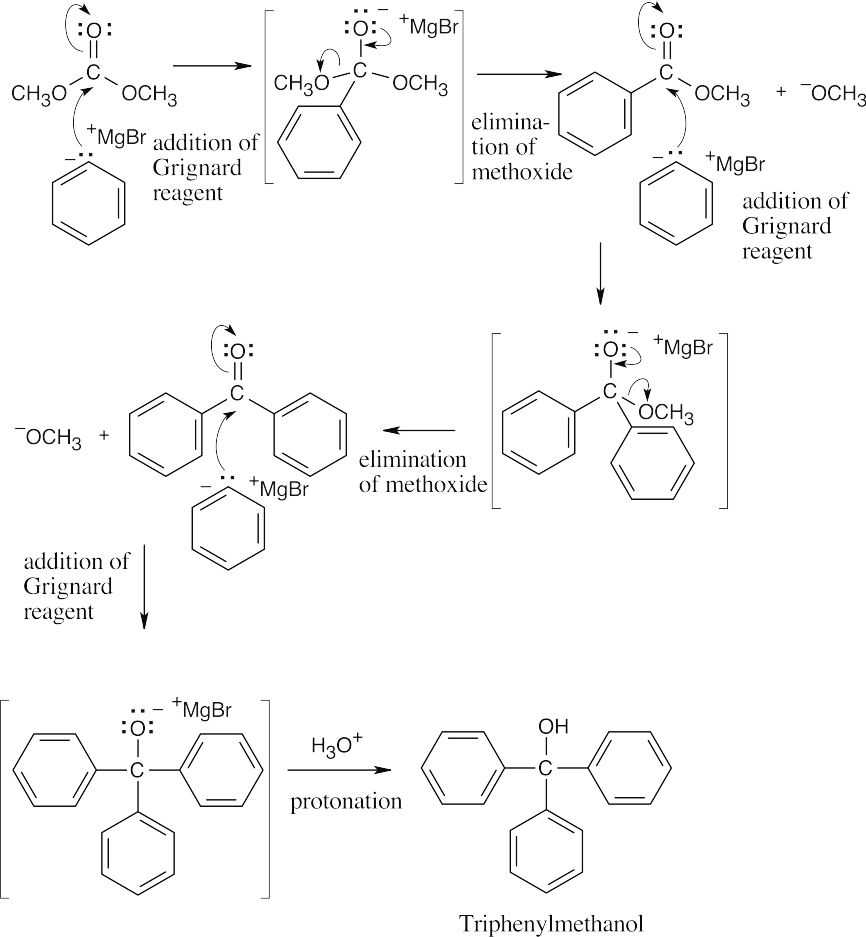
The overall reaction consists of three additions of phenylmagnesium bromide, two eliminations of methoxide and one protonation.
|
21.53 |
(a) |
 |
|
(b) |
 |
|
(c) |
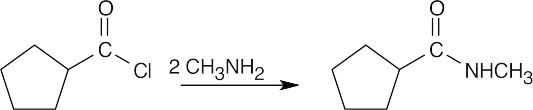 |
|
(d) |
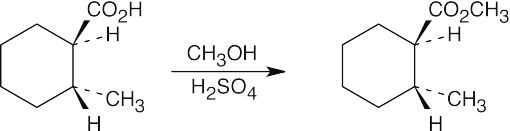 |
|
(e) |
 |
|
(f) |
 |
|
(g) |
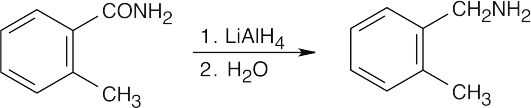 |
|
(h) |
 |
21.54 |
The reactivity of esters in saponification reactions is influenced by steric factors. Branching in both the acyl and alkyl portions of an ester hinders attack of the hydroxide nucleophile. This effect is less dramatic in the alkyl portion of the ester than in the acyl portion because alkyl branching is one atom farther away from the site of attack, but it is still significant.
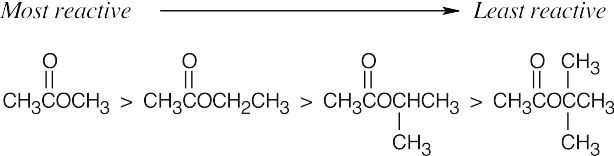
|
21.55 |
2,4,6-Trimethylbenzoic acid has two methyl groups ortho to the carboxylic acid functional group. These bulky methyl groups block the approach of the alcohol and prevent esterification from occurring under Fischer esterification conditions. A possible route to the methyl ester:
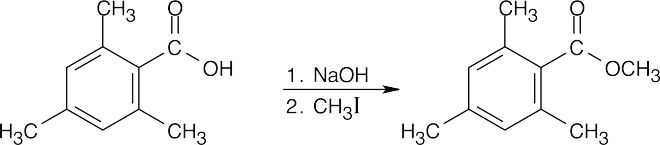
This route succeeds because reaction occurs farther away from the site of steric hindrance. It is also possible to form the acid chloride of 2,4,6-trimethylbenzoic acid and react it with methanol and pyridine.
|
21.57 |
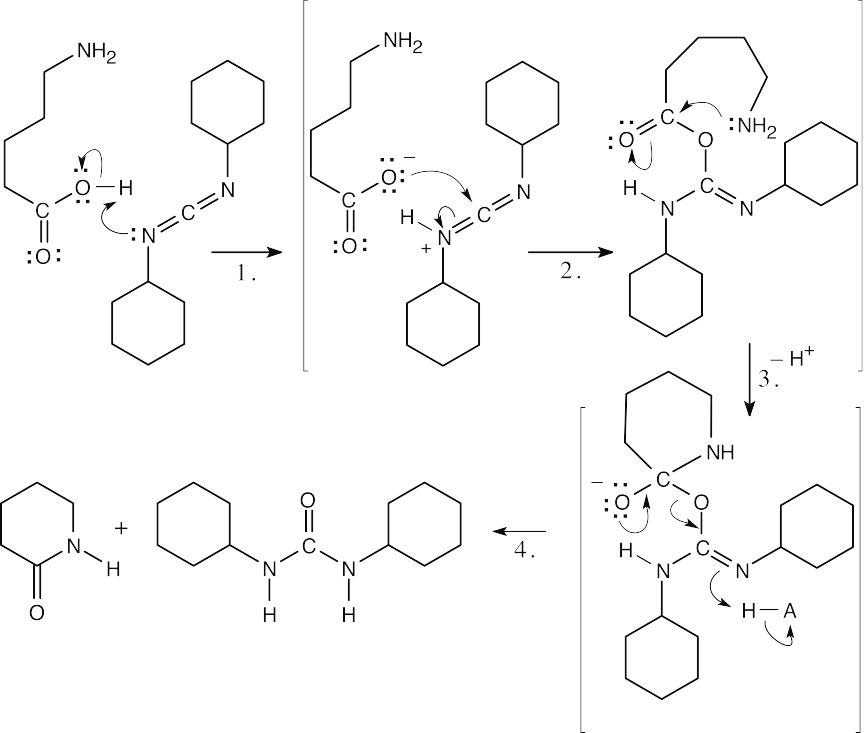 |
|
Step 1: |
The carboxylic acid protonates DCC. |
|
Step 2: |
The carboxylate oxygen adds to DCC to form a reactive intermediate. |
|
Step 3: |
The amine nitrogen adds to the carbonyl group to yield a tetrahedral intermediate. |
|
Step 4: |
The intermediate loses dicyclohexylurea to produce the lactam. |
21.58 |
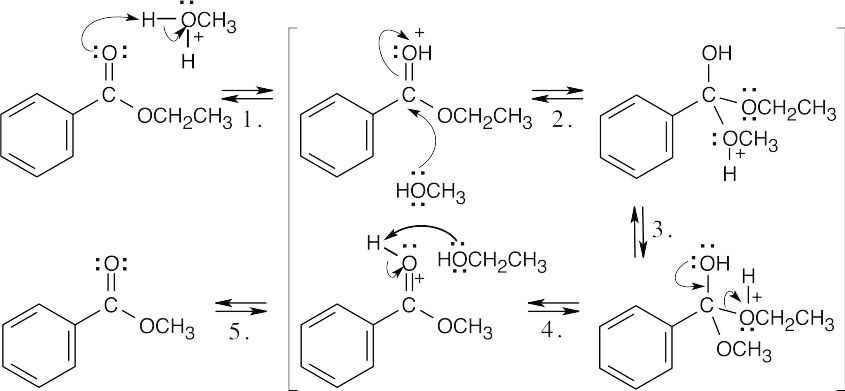 |
|
Step 1: |
Protonation. |
|
Step 2: |
Addition of methanol. |
|
Step 3: |
Proton transfer. |
|
Step 4: |
Loss of ethanol. |
|
Step 5: |
Deprotonation. |
|
In acidic methanol, the ethyl ester reacts by a nucleophilic acyl substitution mechanism to yield a methyl ester. The equilibrium favors the methyl ester because of the large excess of methanol present. |
21.59 |
This reaction is a typical nucleophilic acyl substitution reaction, with azide as the nucleophile and chloride as the leaving group.
|
Step-Growth Polymers
21.60 |
Step 1: |
Water opens the caprolactam ring to form the amino acid intermediate. |
|
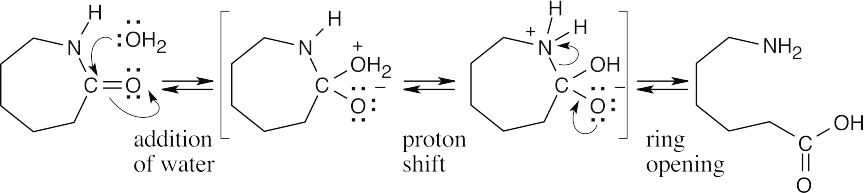 |
|
Step 2: |
Reaction of the intermediate with a second molecule of caprolactam forms a dimer. |
|
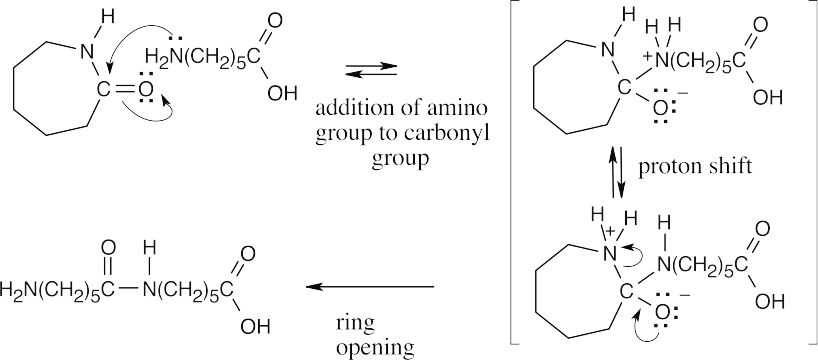 |
|
Step 3 and beyond: |
Reaction of the dimer with caprolactam. This process repeats itself many, many times until the polymer stops growing. Remember that each new bond is formed in a discrete step. Heat forces the equilibrium in the direction of polymer formation. |
|
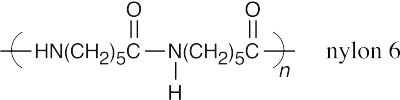 |
21.61 |
Look for the monomer units, which are difunctional compounds, in the polymer.
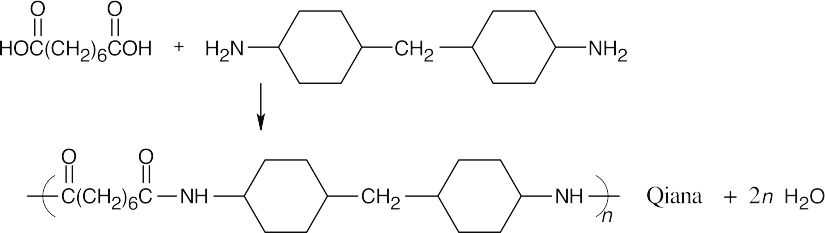
|
21.66 |
(a) |
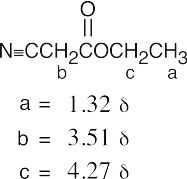 |
(b) |
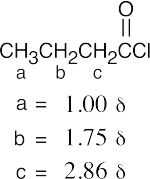 |
General Problems
21.67 |
A negatively charged tetrahedral intermediate is formed when the nucleophile –OH attacks the carbonyl carbon of an ester. An electron-withdrawing substituent can stabilize this negatively charged tetrahedral intermediate and increase the rate of reaction. (Contrast this effect with substituent effects in electrophilic aromatic substitution, in which positive charge developed in the intermediate is stabilized by electron-donating substituents.) Substituents that are deactivating in electrophilic aromatic substitution are activating in ester hydrolysis, as the observed reactivity order shows. The substituents –CN and –CHO are electron-withdrawing; –NH2 is strongly electron-donating. |
Most reactive ⎯⎯⎯⎯⎯⎯⎯⎯⎯⎯⎯⎯⎯⎯⎯⎯⎯⎯⎯⎯⎯⎯→ Least reactive
Y = NO2 > CN > CHO > Br > H > CH3 > OCH3 > NH2
|
21.68 |
The tetrahedral intermediate T can eliminate any one of the three –OH groups to reform either the original carboxylic acid or labeled carboxylic acid. Further reaction of water with mono-labeled carboxylic acid leads to the doubly labeled product.
|
21.69 |
Remember that the 18O label appears in both oxygens of the acetic acid starting material.
|
21.70 |
(a) |
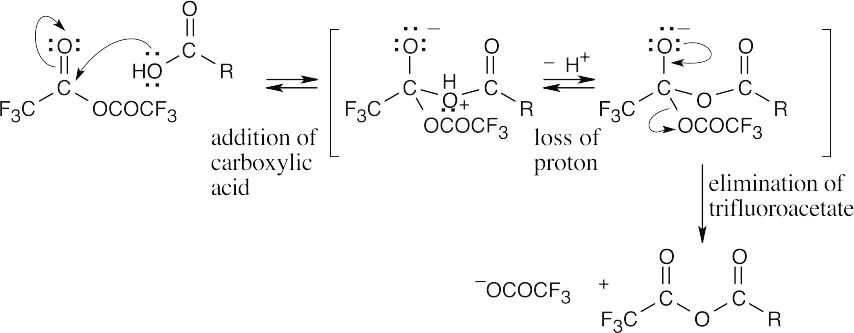 |
|
(b) |
The electron-withdrawing fluorine atoms polarize the carbonyl group, making it more reactive toward nucleophiles. |
|
(c) |
Because trifluoroacetate is a better leaving group than other carboxylate anions, the reaction proceeds as indicated. |
21.71 |
This synthesis requires a nucleophilic aromatic substitution reaction, explained in Section 16.7.
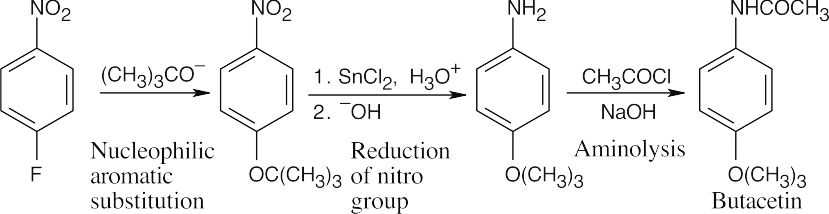
The amide can be formed by the reaction of acetyl chloride with the appropriate amine, which is produced by reduction of the nitro group of the starting material. A nucleophilic aromatic substitution of –F by –OC(CH3)3 can take place because the ring has an electron-withdrawing nitro group para to the site of substitution. Acetic anhydride can also be used to acetylate the amine.
|
21.72 |
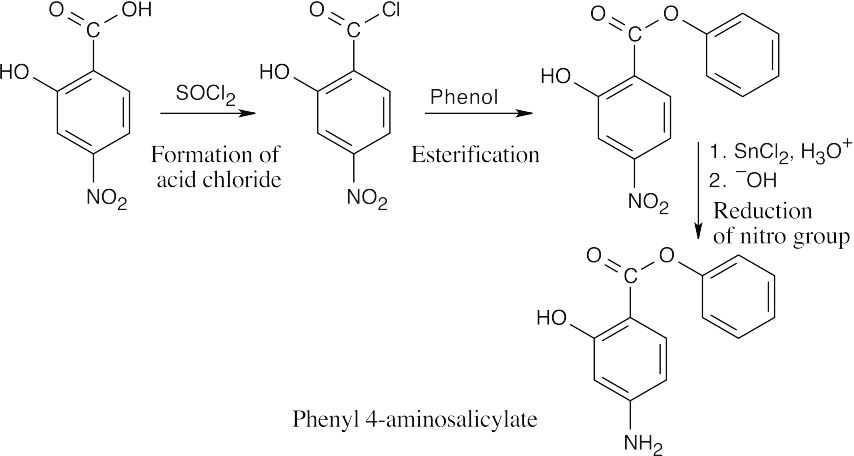 |
21.73 |
Grignard carboxylation yields m-methylbenzoic acid, which can be converted to an acid chloride and treated with diethylamine to produce the amide.
|
21.74 |
Using a rhodium catalyst, the aromatic ring is hydrogenated to form the cis-substituted cyclohexane, which is converted to the trans isomer by heating to 300°. The nitrile starting material is hydrolyzed to form a carboxylic acid, and the methyl group is brominated and treated with ammonia to form the amine.
|
21.75 |
(a) |
Resonance forms show that the carbon of diazomethane is basic, and reaction with an acid can occur to form a methyldiazonium ion.

|
|
(b) |
An SN2 reaction takes place in which the carboxylate ion displaces N2 as the leaving group to form the methyl ester.
|
21.76 |
The product of the reaction of dimethyl terephthalate with glycerol has a high degree of cross-linking and is more rigid than Dacron.
|
21.77 |
(a) |
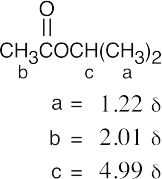 |
(b) |
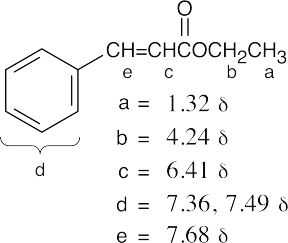 |
21.78 |
(a) |
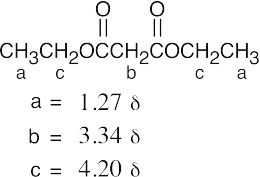 |
(b) |
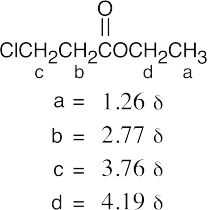 |
21.79 |
Ethyl 4-cyanobenzoate
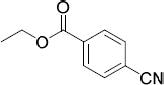
|
21.80 |
Ethyl levulinate (ethyl 4-oxopentanoate)
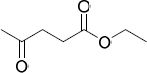
|
21.81 |
Note: The final step in the formation of an amide in each case is deprotonation. The amine that serves as the nucleophile in the reaction will also react as a base and form an unreactive ammonium salt. If a base strong enough to deprotonate the ammonium salt is present, then the amine will be deprotonated and free to react as a nucleophile. The pKa of an ammonium ion is approximately 10. For an acid chloride, the chloride ion is not a strong enough base to deprotonate an ammonium ion (the pKa of HCl is –5), so a second mole of base is needed to complete the reaction. In the case of an ester, an alkoxide leaving group is generated. An alkoxide is a strong enough base to deprotonate an ammonium ion (the pKa of an alcohol is approximately 16). Thus, the reaction does not require any additional base.

|
21.82 |
Addition of the triamine causes formation of cross-links between prepolymer chains.
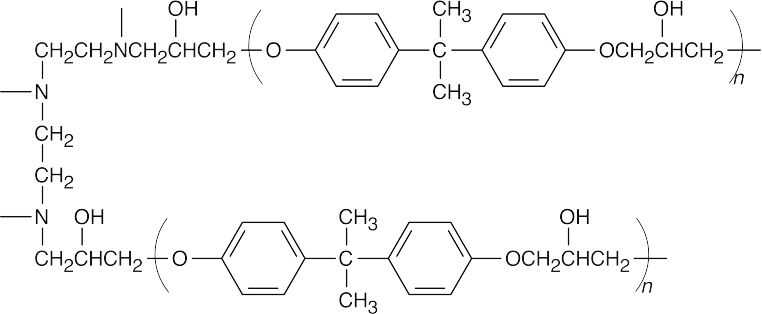
|
This file is copyright 2023, Rice University. All Rights Reserved.