24 Chapter 24 – Amines and Heterocycles Solutions to Problems
Chapter 24 – Amines and Heterocycles
Solutions to Problems
24.1 |
Facts to remember about naming amines:
|
24.2 |
(a) |
(b) |
(c) |
[(CH3)2CH]3N
Triisopropylamine |
(H2C=CHCH2)3N
Triallylamine |
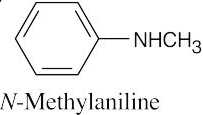 |
(d)
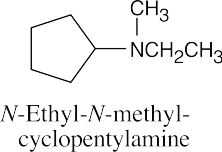
|
(e)
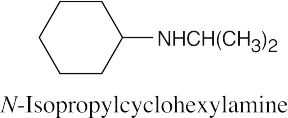
|
(f)
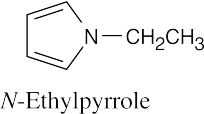
|
|
24.3 |
The numbering of heterocyclic rings is described in Section 24.1.
|
24.4 |
Amines are less basic than hydroxide but more basic than amides. The pKa values of the conjugate acids of the amines in (c) are shown. The larger the pKa, the stronger the base.
|
More Basic |
Less Basic |
(a) |
CH3CH2NH2 |
CH3CH2CONH2 |
(b) |
NaOH |
CH3NH2 |
(c) |
CH3NHCH3
pKa = 10.73 |
pyridine
pKa = 5.25 |
|
24.5 |
The stronger base (propylamine) holds a proton more tightly than the weaker base (benzylamine). Thus, the propylammonium ion is less acidic (larger pKa) than the benzylammonium ion (smaller pKa).
To calculate pKb: Ka · Kb = 10 – 14, pKa + pKb = 14 and pKb = 14 – pKa. |
24.6 |
The basicity order of substituted arylamines is the same as their reactivity order in electrophilic aromatic substitution reactions because, in both cases, electron-withdrawing substituents make the site of reaction more electron-poor and destabilize a positive charge.
|
24.7 |
Use the expressions shown in Section 24.5.
At pH = 7.3, virtually 100% of the pyrimidine molecules are in the neutral form. |
24.8 |
Amide reduction can be used to synthesize most amines, but nitrile reduction can be used to synthesize only primary amines. Thus, the compounds in (b) and (d) can be synthesized only by amide reduction.
The compounds in parts (b) and (d) can’t be prepared by reduction of a nitrile. |
24.9 |
Step 1: Addition of hydroxide.
Step 2: Ring opening.
Step 3: Proton transfer.
Step 4: Addition of hydroxide.
Step 5: Elimination of amine.
Step 6: Proton transfer. |
24.10 |
The upper reaction is the azide synthesis, and the lower reaction is the Gabriel synthesis.
|
24.11 |
Look at the target molecule to find the groups bonded to nitrogen. One group comes from the aldehyde/ketone precursor, and the other group comes from the amine precursor. In most cases, two combinations of amine and aldehyde/ketone are possible.
|
24.13 |
In both of these reactions, the product amine is formed from a carboxylic acid derivative precursor that has one more carbon than the amine. In the Hofmann rearrangement, the precursor is an amide, which is treated with Br2, NaOH and H2O. In the Curtius rearrangement, the precursor is an acid chloride, which is treated with NaN3, then with H2O and heat.
(a)

|
(b)
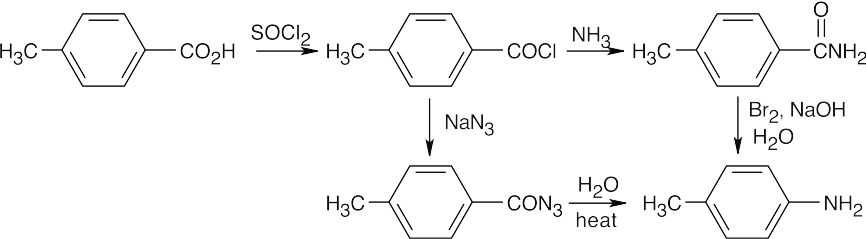
|
|
24.14 |
The Hofmann elimination yields alkenes and amines from larger amines. The major alkene product has the less substituted double bond, but all possible products may be formed. The hydrogens that can be eliminated are starred. When possible, cis and trans double bond isomers are both formed.
(a)
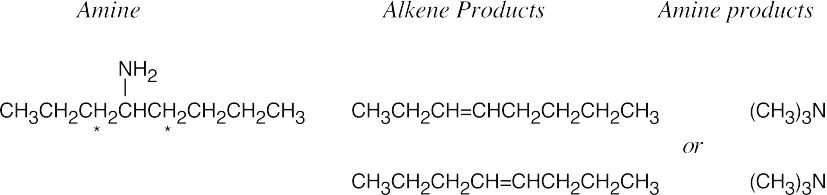
Both hydrogens that might be eliminated are secondary, and both possible products should form in approximately equal amounts. |
(b)

|
(c)

In each of the above reactions, only one product can form. |
(d)
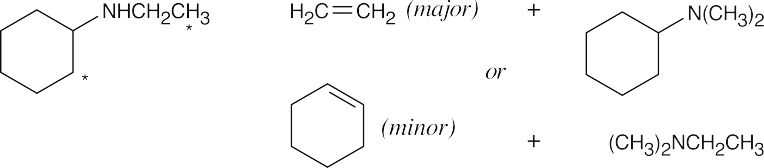
The first pair of products in (d) results from elimination of a primary hydrogen and are the major products. The second pair of products results from elimination of a secondary hydrogen. |
|
24.15 |
The product, which contains both the double bond and the tertiary amine in an ring-opened structure, can undergo a second Hoffmann elimination.

|
|
24.16 |
This reaction sequence is similar to the sequence used to synthesize sulfanilamide. Key steps are: (1) treatment of aniline with acetic anhydride to modulate reactivity, (2) reaction of acetanilide with chlorosulfonic acid, (3) treatment of the chlorosulfonate with the heterocyclic base, and (4) removal of the acetyl group.
|
24.17 |
In all of these reactions, benzene is nitrated and the nitro group is ultimately reduced, but the timing of the reduction step is important in arriving at the correct product. In (a), nitrobenzene is immediately reduced and alkylated. In (c), chlorination occurs before reduction so that chlorine can be introduced in the m-position. In (b) and (d), nitrobenzene is reduced and then acetylated in order to overcome amine basicity and to control reactivity. In both cases, the acetyl group is removed in the last step.
Either method of nitro group reduction (SnCl2, H2) can be used in all parts of this problem; both methods are shown.
(a)

Mono- and trialkylated anilines are also formed. |
(b)
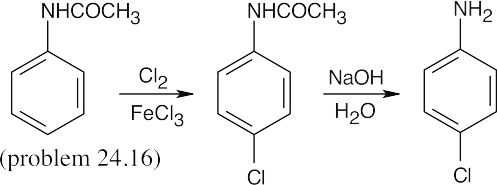
|
(c)

|
(d)
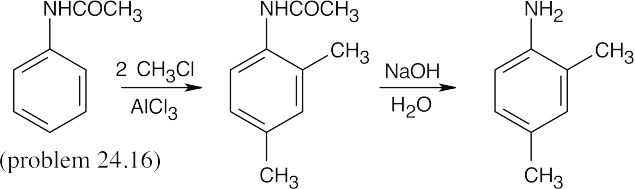
|
|
24.18 |
(a)
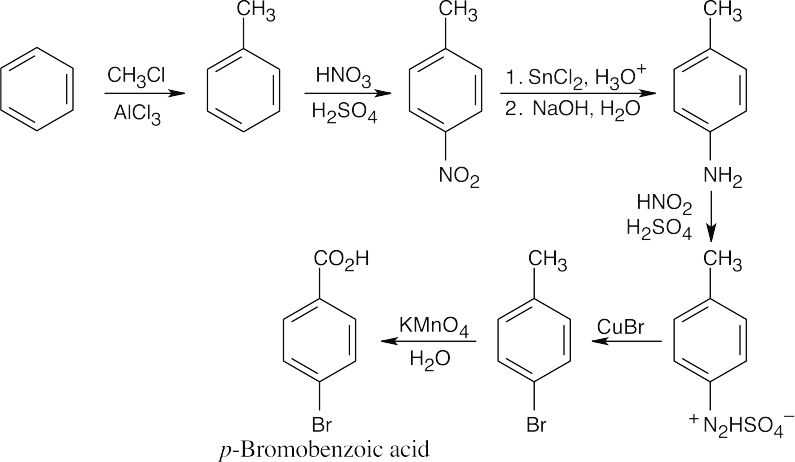
The route shown above is one of several ways to synthesize p-bromobenzoic acid and is definitely not the simplest way. (The simplest route is Friedel–Crafts alkylation → bromination → oxidation). The illustrated synthesis shows the use of the diazonium replacement reaction that substitutes bromine for a nitro group. Oxidation of the methyl group yields the substituted benzoic acid. |
(b)
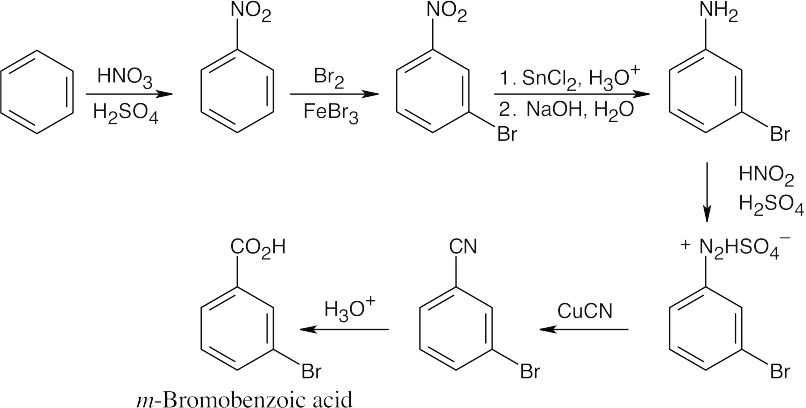
Again, this isn’t the easiest route to this compound. In this case, nitration is followed by bromination, then by diazotization, treatment with CuCN, and hydrolysis of the nitrile. |
(c)
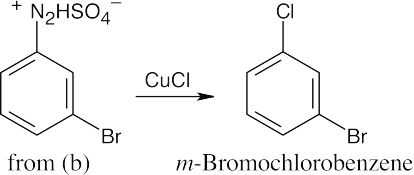
|
(d)
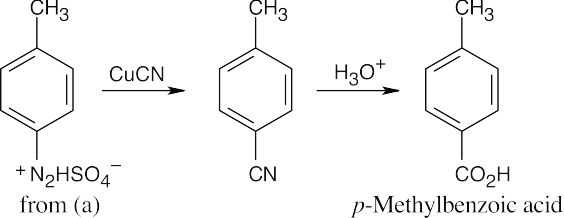
|
(e)
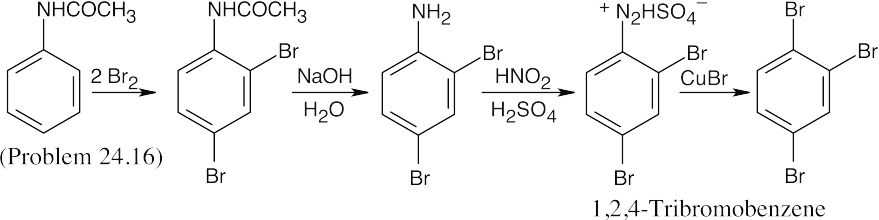
|
|
24.19 |
Coupling takes place between N,N-dimethylaniline and a benzenediazonium salt to yield the desired product. |
24.20 |
Thiazole contains six π electrons. Each carbon contributes one electron, nitrogen contributes one electron, and sulfur contributes two electrons to the ring π system. Both sulfur and nitrogen have lone electron pairs in sp2 orbitals that lie in the plane of the ring. |
24.21 |
4.1% of histidine molecules have the imidazole nitrogen in the protonated form at the physiological pH |
24.22 |
Reaction at C3 is favored over reaction at C2 or C4. The positive charge of the cationic intermediate of reaction at C3 is delocalized over three carbon atoms, rather than over two carbons and the electronegative pyridine nitrogen as occurs in reaction at C2 or C4. |
24.23 |
The side chain nitrogen atom of N,N-dimethyltryptamine is more basic than the ring nitrogen atom because its lone electron pair is more available for donation to a Lewis acid. The aromatic nitrogen electron lone pair is part of the ring π electron system. |
24.24 |
Positive charge can be stabilized by the nitrogen lone-pair electrons in reaction at both C2 and C3. In reaction at C2, however, stabilization by nitrogen destroys the aromaticity of the fused benzene ring. Reaction at C3 is therefore favored, even though the cationic intermediate has fewer resonance forms, because the aromaticity of the six-membered-ring is preserved. |
24.25 |
The IR spectrum (pair of bands at around 3300 cm–1) shows that B is a primary amine, and the 1H NMR spectrum shows a 9-proton singlet, a one-proton quartet, and a 3-proton doublet. An absorption due to the amine protons is not visible. |
Additional Problems
Visualizing Chemistry
24.28 |
Hofmann elimination is an E2 elimination, in which the two groups to be eliminated must be 180° apart. The product that results from this elimination geometry is the Z isomer. |
24.29 |
The indicated nitrogen is most basic because it is more electron-rich. The electrons of the other nitrogens are part of the fused-ring π system and are less available for donation to a Lewis acid. |
Mechanism Problems
24.30 |
(a) |
Mechanism:
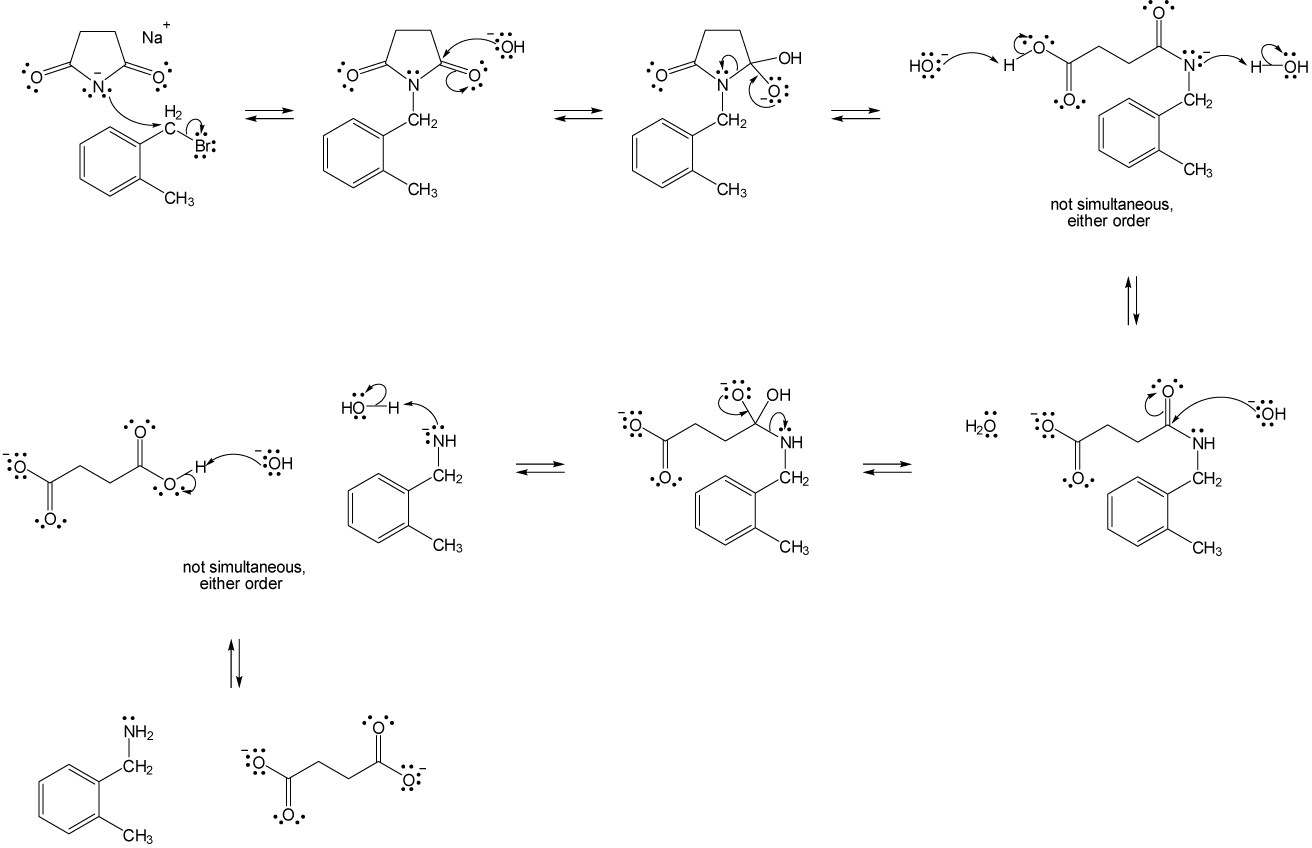
|
(b) |
Mechanism:
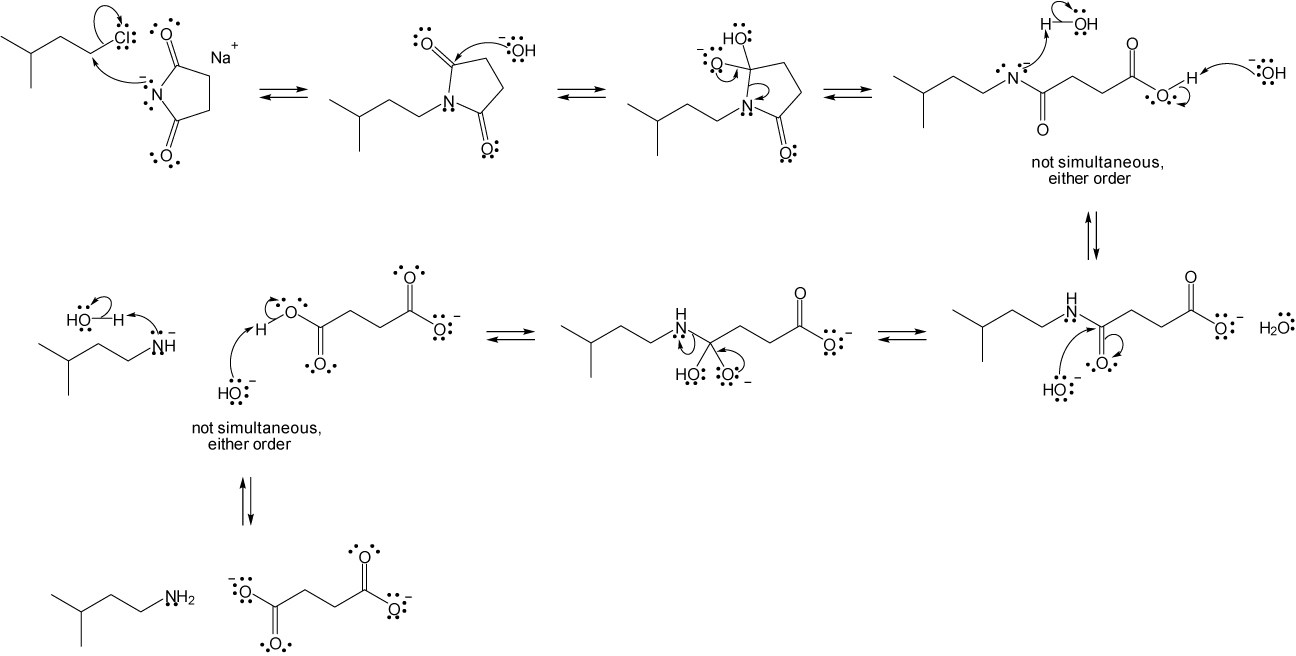
|
|
24.31 |
(a) |
Mechanism:
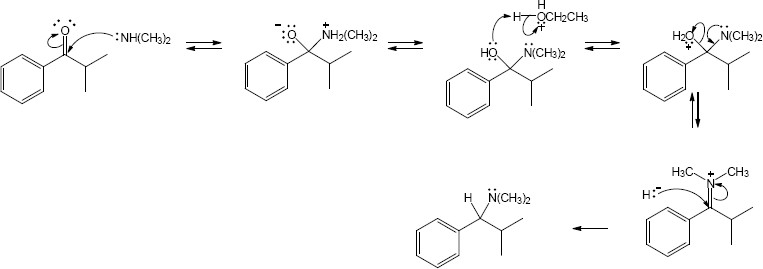
|
(b) |
Mechanism:
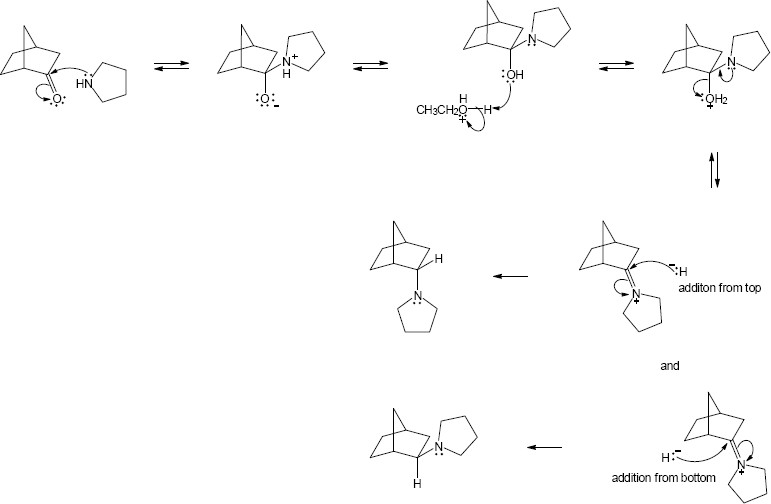
|
|
24.32 |
(a) |
Mechanism:
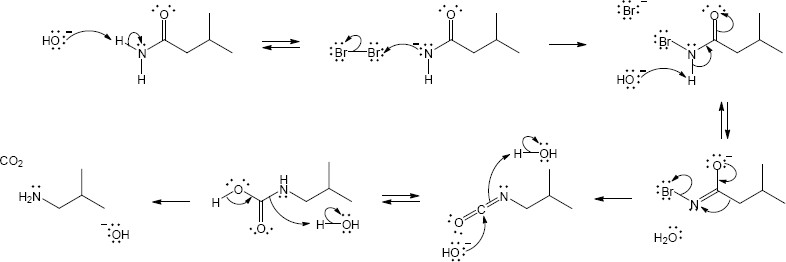
|
(b) |
Mechansim:
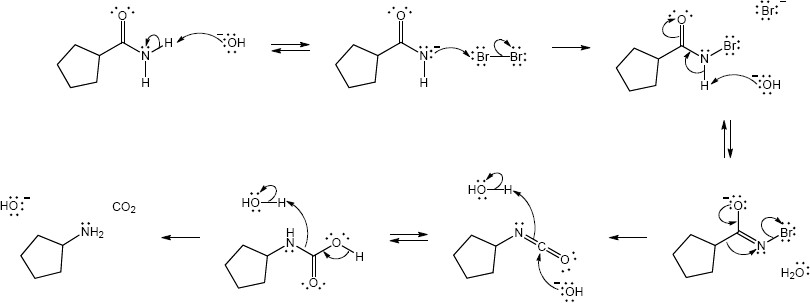
|
(c) |
Mechanism:
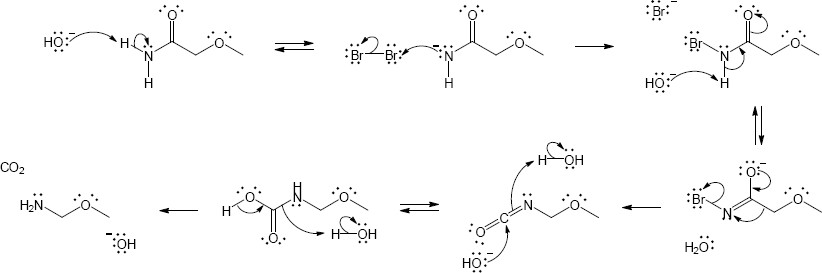
|
(d) |
Mechanism:
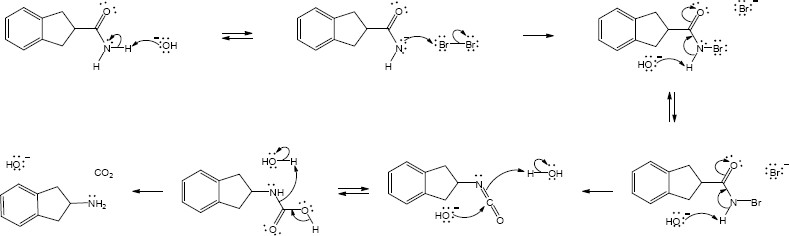
|
|
24.33 |
(a) |
Mechanism:
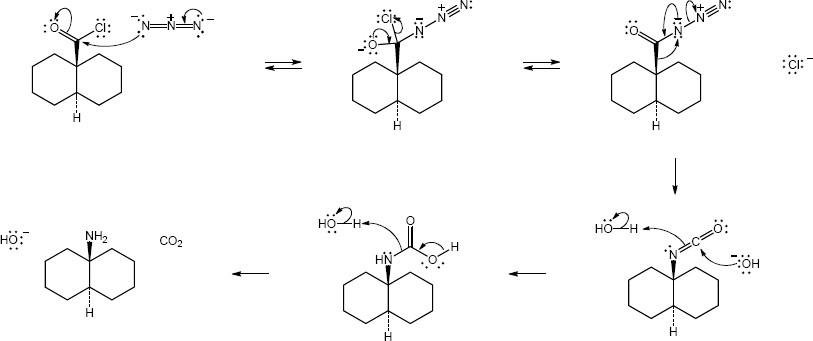
|
(b) |
Mechanism:
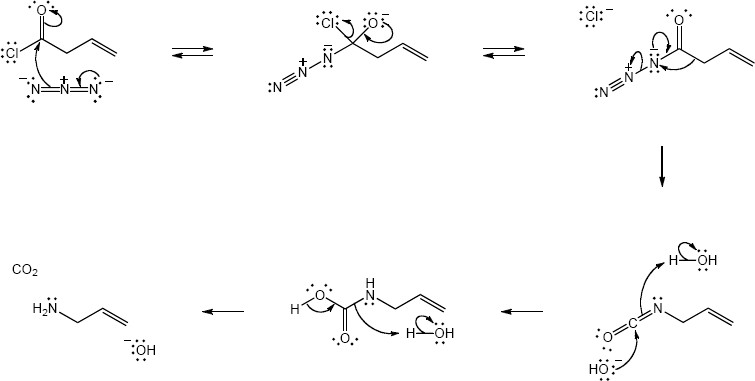
|
|
24.35 |
Steps 1, 6: Protonation.
Steps 2, 7: Nucleophilic addition.
Steps 3, 8: Proton transfer.
Steps 4, 9: Loss of water.
Steps 5, 10: Loss of proton.
The mechanism consists of the nucleophilic addition of ammonia, first to one of the ketones, and then to the other, with loss of two equivalents of water. |
24.36 |
Steps 1, 6: Protonation.
Steps 2, 7: Nucleophilic addition of hydroxylamine.
Steps 3, 8: Proton transfer.
Steps 4, 9: Loss of water.
Steps 5, 10: Loss of proton.
This mechanism is virtually identical to the mechanism illustrated in the previous problem and involves two nucleophilic additions to carbonyl groups, with loss of water. These addition-eliminations are nucleophilic acyl substitution reactions. |
24.37 |
Benzaldehyde first reacts with methylamine and NaBH4 in the usual way to give the reductive amination product N-methylbenzylamine. This product then reacts further with benzaldehyde in a second reductive amination to give N-methyldibenzylamine.
|
24.38 |
Step 1: Nucleophilic addition.
Step 2: Cyclization.
Step 3: Elimination of lysine.
Step 4: Elimination of the other lysine.
Step 5: Tautomerization. |
24.39 |
The reaction of trimethylamine with ethylene oxide is an SN2 reaction that opens the epoxide ring.
|
24.40 |
Steps 1 – 3: E1 elimination (protonation, loss of HOCH3, deprotonation).
Steps 4 – 6: SN2 substitution (protonation, substitution, deprotonation).
Step 7: E1 elimination of carbamate.
Steps 8 – 9: Conjugate addition of DNA (addition, deprotonation).
Notice that five of the nine steps are either protonations or deprotonations. |
24.41 |
Step 1: Addition of NH3.
Step 2: Elimination of –OH.
Step 3: Addition of –CN.
Step 4: Hydrolysis of nitrile.
The mechanism of acid-catalyzed nitrile hydrolysis is shown in Problem 20.25. |
24.42 |
Step 1: Conjugate addition of hydrazine.
Step 2: Proton transfer.
Step 3: Nucleophilic acyl substitution, forming the cyclic amide.
Step 4: Proton transfer. |
24.43 |
Hofmann rearrangement (the mechanism is shown in Section 24.6) of an α-hydroxy amide produces a carbinolamine intermediate that expels ammonia to give an aldehyde. |
24.44 |
Step 1: Conjugate addition of amine.
Step 2: Proton transfer.
Step 3: Nucleophilic addition of amine.
Step 4: Proton transfer.
Step 5: Elimination of methanol. |
24.45 |
Step 1: SN2 displacement of Br– by amine.
Step 2: Deprotonation.
Step 3: Conjugate addition of alcohol.
Step 4: Proton transfer. |
24.46 |
Step 1: Nucleophilic addition of the amine to the protonated aldehyde.
Step 2: Proton transfer.
Step 3: Loss of water.
Step 4: Electrophilic aromatic substitution.
Step 5: Loss of proton. |
Naming Amines
Amine Basicity
20.50 |
The pyrrole anion, C4H4N:–, is a 6 π electron species that has the same electronic structure as the cyclopentadienyl anion. Both of these anions possess the aromatic stability of 6 π electron systems. |
24.51 |
The “a” nitrogen is most basic because its electron pair is most available to Lewis acids.
The “c” nitrogen is the least basic because the lone-pair electrons of the pyrrole nitrogen are part of the ring π electron system. |
24.52 |
The inductive effect of the electron-withdrawing nitro group makes the amine nitrogens of both m-nitroaniline and p-nitroaniline less electron-rich and less basic than aniline.
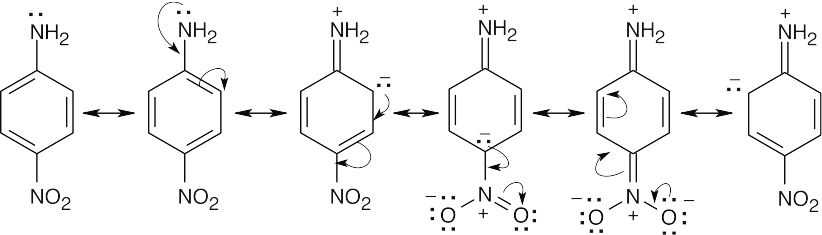
When the nitro group is para to the amino group, conjugation of the amino group with the nitro group can also occur. p-Nitroaniline is thus even less basic than m-nitroaniline. |
|
Synthesis of Amines
24.56 |
First, synthesize aniline from benzene, as shown in Problem 24.55 (a).
|
24.57 |
(a)

|
(b)

|
(c)

|
(d)

|
(e)

|
(f0

|
(g)
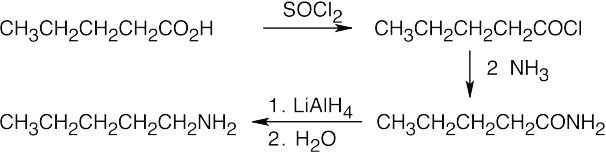
|
|
Reactions of Amines
24.61 |
(a)

|
(b)

|
(c)

|
(d)

|
(e)

|
(f)

|
(g)

|
(h)

|
In (e), the amine reacts with the Grignard reagent and inactivates it. |
24.62 |
Hydrogens that can be eliminated are starred. In cases where more than one alkene can form, the alkene with the less substituted double bond is the major product.
|
Spectroscopy
24.65 |
The 1H NMR of the amine shows five peaks. Two are due to an ethyl group bonded to an electronegative element (oxygen), two are due to four aromatic ring hydrogens, and the peak at 3.40 δ is due to two amine hydrogens.
|
General Problems
24.68 |
(a) NH3, NaBH4;
(b) excess CH3I;
(c) Ag2O, H2O, heat;
(d) RCO3H
(e) (CH3)2NH.
Step (e) is an SN2 ring opening of the epoxide by nucleophilic substitution of the amine at the primary carbon. |
24.69 |
Oxazole is an aromatic 6 π electron heterocycle. Two oxygen electrons and one nitrogen electron are in p orbitals that are part of the π electron system of the ring, along with one electron from each carbon. An oxygen lone pair and a nitrogen lone pair are in sp2 orbitals that lie in the plane of the ring. Since the nitrogen lone pair is available for donation to acids, oxazole is more basic than pyrrole. |
24.70 |
Protonation occurs on oxygen because an O-protonated amide is stabilized by resonance. |
24.72 |
(a) CH2=CHCH2Cl, AlCl3;
(b) Hg(OAc)2, H2O; NaBH4;
(c) CrO3, H3O+;
(d) CH3NH2, NaBH4. |
24.73 |
The last step of the synthesis is a reductive amination of a ketone that is formed by oxidation of the corresponding alcohol. The alcohol results from the Grignard reaction between cyclopentylmagnesium bromide and propylene oxide. |
24.74 |
(a)
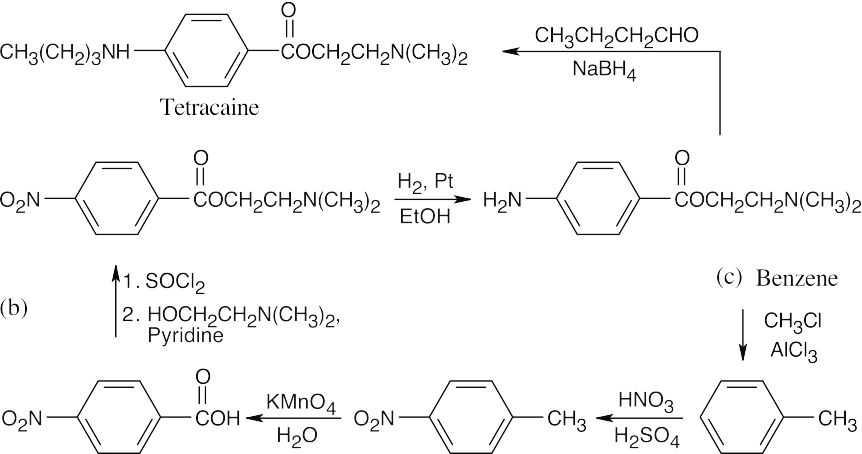
|
The synthesis in (a) is achieved by a reductive amination reaction. Reactions in (b) include formation of an acid chloride, esterification, and reduction of the nitro group. |
24.75 |
We know the location of the –OH group of tropine because it is stated that tropine is an optically inactive alcohol. This hydroxyl group results from basic hydrolysis of the ester that is composed of tropine and tropic acid. |
24.76 |
Tropilidene results from two cycles of Hofmann elimination on tropidene. |
24.77 |
The formula C9H17N indicates two degrees of unsaturation in the product. Both are probably due to rings since the product results from catalytic reduction.
Step 1: Reduction of nitrile
Step 2: Nucleophilic addition.
Step 3: Dehydration.
Step 4: Reduction of double bond. |
24.78 |
The molecular formula indicates that coniine has one double bond or ring, and the Hofmann elimination product shows that the nitrogen atom is part of a ring.
|
24.79 |
Step 1: Michael addition.
Step 2: Ester hydrolysis; decarboxylation.
Step 3: Reduction.
Step 4: Cyclization.
Step 5: Reduction. |
24.80 |
When you see –CH2NH2, think of the reduction of a nitrile. The nitrile comes from substitution of a benzylic bromide by –CN. |
24.81 |
(a)

|
(b)

|
The reactive intermediate is benzyne, which undergoes a Diels–Alder reaction with cyclopentadiene to yield the observed product. |
24.82 |
Two successive cycles of Hofmann elimination lead to formation of cyclooctatriene.
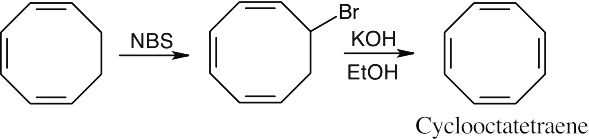
Allylic bromination followed by elimination yield cyclooctatetraene. |
|
24.83 |
(a) |
(b) |
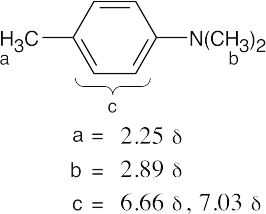 |
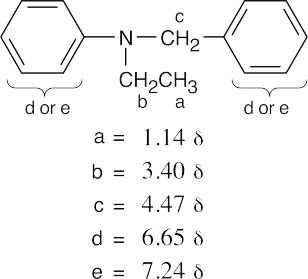 |
|
24.84 |
Note: Although there are several resonance forms, with the exception of the completely neutral form, the most stable one is the charge separated form with a negative charge on the pyridine nitrogen. The increased electron density on the pyridine nitrogen increases its ability to react as a nucleophile.
|
This file is copyright 2023, Rice University. All Rights Reserved.