Chapter 1: Adaptation and Evolution
Vocabulary
- Acclimation: process in which an individual organism adjusts its phenotype in response to a change in its environment (reversible process)
- Adaptation: morphological, behavioral, and physiological traits that increase fitness in a particular environment
- Adaptive Radiation: diversification of strategies from other organisms to fill an ecological niche
- Allele: different forms of a gene
- Alpha diversity: a metric to quantify the diversity of types of organisms and/or their relative abundance
- Anthropogenic: caused or influenced by human activity
- Beta diversity: a metric to quantify the variation (difference) between communities
- Cline: a measurable, gradual change over a geographic region in the average of some phenotypic character, such as size and coloration
- Common Garden Experiment: Rear offspring with different genotypes under the same environmental condition
- Determines genetic component to phenotype
- Complete Dominance: completely masks the recessive allele in heterozygotes
- Developmental Plasticity: the ability of an organism to adjust its phenotype in response to environmental cues during early stages of development. Changes are permanent.
- Directional Selection: over time, population shifts to either higher or lower extremes
- Disruptive Selection: over time, population shifts to BOTH extremes and does not favor intermediate
- Ecotype: subpopulation of a species that is adapted to a specific environment
- Fitness: the ability of an organism / population / species to survive and reproduce (and thus contribute genes to the next generation) in a specific environment
- Gamma diversity: the overall species diversity across communities within a larger geographic area
- Genetic Differentiation: differences in allele frequencies within or between populations or subpopulations
- Genotype: set of alleles in DNA that influence phenotype
- Heterozygous: two different alleles for a gene
- Homozygous: two of the same alleles for a gene
- Incomplete Dominance: does not completely mask the recessive allele in heterozygotes; a combination of parental phenotypes are present
- Locus: the actual fixed position of the gene within the chromosome. (plural: loci)
- Microbiome: refers to community as a collection of genomes (DNA)
- Microbiota: refers to collection of microbes (living organisms) in a community
- Natural Selection: the mechanism of evolution; environmental pressures result in differential survival and reproduction rates, causing different phenotypes (and adaptations) to become more prevalent in a population
- Requires variation and heritability that leads to differences in fitness
- Norm of Reaction: a quantitative measure of phenotypic plasticity, calculated as the range in phenotype variation from the same genotype in different environments
- A greater norm of reaction will have greater phenotypic variation
- Dramatic differences in phenotype will graph as a steep slope
- A differing environment results in a differing phenotype
- Use common garden and reciprocal transplant experiments to determine norm of reaction
- Phenotype: the observable / physical traits of an organism that result from the interaction of the organism’s genotype and the environment
- Phenotypic Plasticity: single genotype gives rise to different phenotypes based on environmental conditions (e.g., temperature). Could result from acclimation (reversible) or developmental plasticity (permanent)
- Phenotypic plasticity in itself is a heritable trait (plasticity with respect to a given trait)
- Qualitative Traits: a trait that fits into a specific category; involves descriptive qualities. Some examples of qualitative traits include blood type, coat color, presence/absence of a tail
- Quantitative Traits: a trait on a spectrum of values; numerical categories. Some examples of quantitative traits include height and blood pressure
- Reciprocal Transplant Experiment: swap the conditions under which two different ecotypes are reared
- Determines genetic component to phenotype
- Stabilizing Selection: over time, population selects for an intermediate phenotype
- Subpopulation: a subset of a larger population
- Local population of interbreeding individuals
- a species is essentially a genetic “gradient” of (sub)populations, each of which are slowly evolving over time
- Symbiosis: long-term relationship between two different species
Outline
Natural Selection changes gene frequency by favoring different phenotypes.
- Acts on individual variation and results in population-level impacts.
- Requires variation:
- Variation must be heritable.
- Result in differences in survival and reproduction rates through environmental interactions.
- Environmental interactions are selective agents.
- Different environments have different selective pressures:
- Examples: nutritional landscape, geography, vegetation availability, substrate availability, weather, salinity, oxygen levels, temperature, presence of predators
- Predation can be context dependent and vary among individuals
- Variation must be heritable.
Fitness = The ability to successfully create fertile offspring
- Individuals with higher fitness tend to survive longer and produce more offspring compared to other individuals in their population, at a given time.
- Because they have traits that are better suited to the prevailing conditions, the fittest individuals have higher reproductive success (i.e., produce more offspring), and therefore pass their traits to the next generation at a higher proportion.
- Note that this is different from r-selected species (discussed in Chapter 2), which tend to invest more energy in producing more offspring per clutch (or brood, or reproductive season), compared to other species.
- In this way, evolution results from adaptations to conditions in a given environment at a given time. There is no “better” or “worse” end point, especially since environments are constantly changing.
Other Evolutionary Processes:
Evolutionary Process: | Definition: | Change to Genetic Variation: |
Bottleneck Effect | A sudden decrease in population size or allele frequency, causing a “bottleneck”. Bottlenecks occur when most of the original population dies off or leaves an area. | Decrease |
Founder Effect | A small number of “founder” individuals colonize a new area, whose offspring have reduced genetic diversity. The new founder population is less genetically diverse because it is built off of a small off-shoot of the original source population (which remains intact). | Decrease (when compared to original/source population) |
Mutation | Changes in the sequence of an organism’s DNA. May be caused by a nucleotide insertion, deletion, or substitution; or by environmental radiation or chemical exposure. Mutations contribute to genetic variation (and, thus, to natural selection). | Increase |
Genetic Drift | Change in allele frequencies as a result of random chance. Genetic drift can cause gene variants to disappear. | Decrease |
Sexual Reproduction | The combination of genetic information from two individuals. | Increase |
Migration | Movement of individuals between populations. | Decrease in variation for the source population that loses individuals
Increase in variation for the sink population that gains individuals |
Gene Flow | Movement of genes between populations. | Decrease in the differences between populations.
Without gene flow, different alleles are more likely to become “fixed” in different populations. Over time, accumulating genetic differences contribute to speciation. |
Artificial Selection | The selection of and breeding for desirable traits in organisms by humans. | Decrease if humans select for / against one specific trait. (e.g., harvesting deer with larger antlers)
Increase when we breed several varieties with distinct characteristics. (For example, consider the variation among dog breeds – chihuahuas, poodles, great Danes, etc. – or the range of sizes and colors of tomatoes) |
Read through the figure below for examples of several evolutionary processes at work in conservation management.
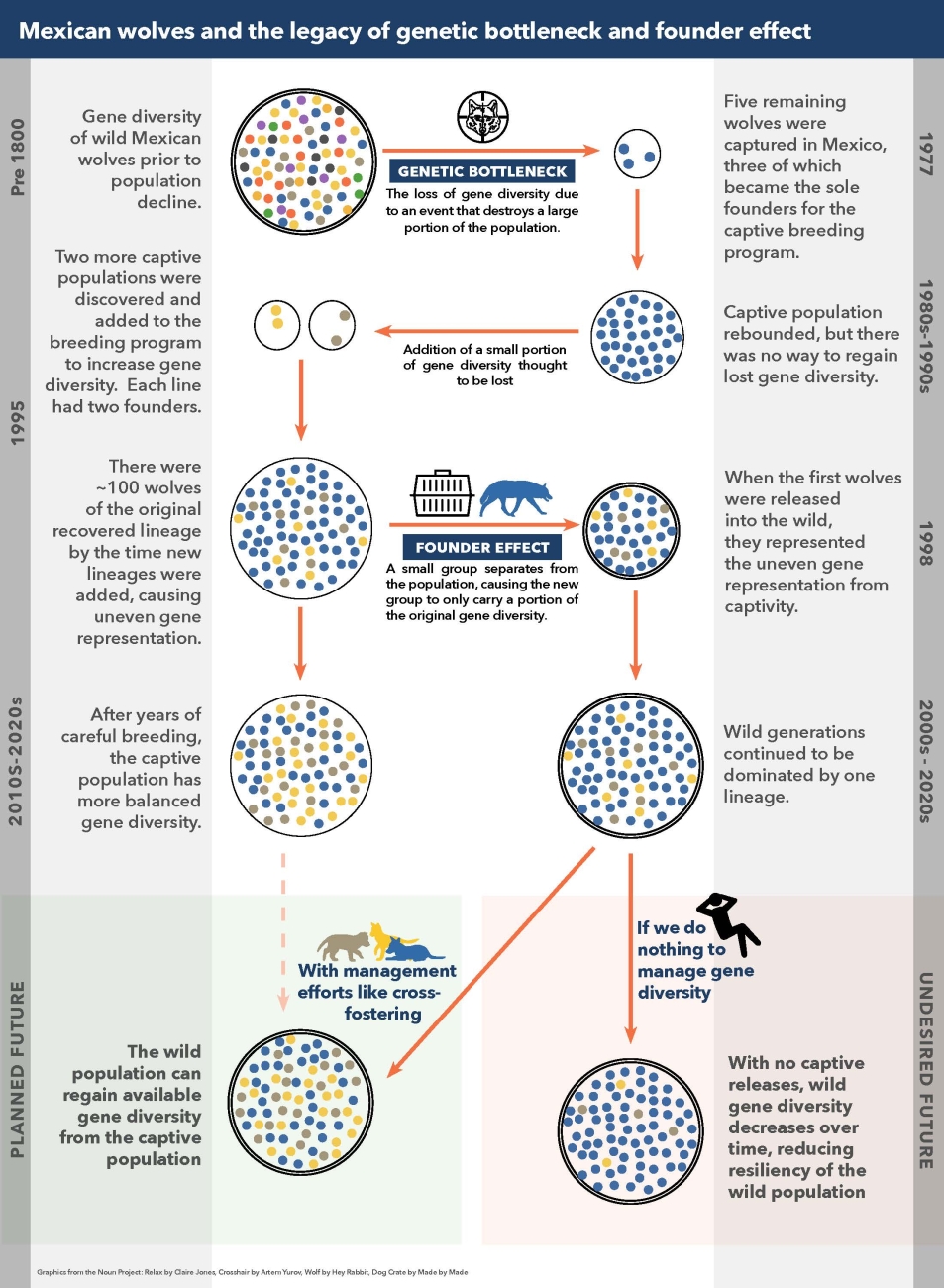
Assortative Mating:
- Individuals choose mates based on phenotype (i.e., desirable characteristics)
- Assortative mating changes genotype frequencies, but not allele frequencies (under the assumption that all individuals that can mate are mating in a population). Two types:
- Positive: Mates are phenotypically more similar to each other than expected by chance.
- Negative: Mates are phenotypically less similar to each other than expected by chance (results in an increase in the frequency of heterozygotes).
Inbreeding:
Mating of individuals in the population that are more closely related than expected by random chance.
- Increases homozygosity at all loci.
- Can result in decreased fertility, loss of vigor, reduced fitness, reduced pollen and seed fertility in plants, and even death (these consequences are known as inbreeding depression).
Inbreeding vs Positive Assortative Mating:
Inbreeding: | Assortative Mating: |
Affects all loci | Affects only loci that control for similar phenotypic characteristics |
ONLY Natural Selection leads to adaptation (as a result of its connection to environmental pressure).
- Response to specific environmental pressure that results in a difference in fitness
- Sexual & artificial selection are caused by internal forces
Question from a Student:
“I still don’t really get why sexual selection does not lead to adaptation. I understand that it doesn’t lead to adaptation of traits that increase fitness with respect to environmental factors but it still seems to me that it would lead to adaptation for sexually preferred traits (it still would lead to selection based on an ability to reproduce). Same with artificial selection, but with adaptation with respect to anthropocentric changes. For instance, adaptation to global climate change would be an artificial selection (unless I am misunderstanding the context under which we are using the term artificial selection) but it would still be adaptation.”
Answer: Great question! Let’s start with the definition.
Adaption happens when a genetically determined characteristic improves an organism’s ability to survive and reproduce under prevailing environmental conditions.
This means that any fitness that is not associated with the environment cannot be adaptation.
- While sexual selection may still result in a change in gene frequency over time, it’s a change driven by mates’ preferences, not by environmental conditions. (Yes, sexual selection can be influenced by physical characteristics that are deemed more desirable; but these are only based on the individual’s preferences, and not by external factors.)
- That is also why domestic breeding is referred to as “artificial selection”: even though dogs, cats, and cattle are wildly successful at passing genes on to future generations, humans (generally) select specific individuals to breed, so mating pairs and their offspring are a product of human decision making instead of those individuals’ success in their environment.
- Note: Artificial selection (i.e., human-directed breeding) is different from global climate change and other anthropogenic changes that affect environmental conditions. As environmental conditions (e.g., temperature, precipitation, resource availability) change, they select for different heritable traits – meaning that species do adapt and evolve in response to anthropogenic change.
Types of Selection:
Stabilizing Selection: | Directional Selection: | Disruptive Selection: |
Over time, population selects for an intermediate phenotype
→ Selection against both extremes |
Over time, population shifts to either higher or lower extremes
→ Selection against one extreme |
Over time, population shifts to BOTH extremes and does not favor intermediate
→ Selection against the mean |
![]() |
![]() |
![]() |
Adaptation is Context Dependent:
- Traits which increased an organism’s fitness may not remain beneficial under changing conditions.
- An adaptation to one selective pressure may not increase fitness in later generations.
Phenotypic Plasticity:
- Acclimation = reversible
- Process in which an individual organism adjusts to a change in its environment (reversible process).
- Developmental plasticity = irreversible
- May incur similar changes to acclimation, but these responses to the environment occur at early stages of an organism’s development and they are permanent.
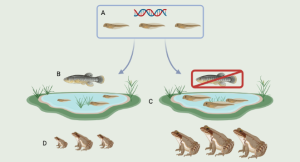
- Testing Plasticity of Traits:
- Common Garden Experiment: Rear offspring with different genotypes under the same environmental condition.
- Determines genetic components to phenotype by controlling for environmental influences.
- Reciprocal Transplant Experiment: Swap the conditions under which two different ecotypes are reared.
- Determines the genetic and environmental effects on phenotype while also detecting for local adaptation
- Common Garden Experiment: Rear offspring with different genotypes under the same environmental condition.
Norm of reaction:
- The range of particular phenotypes for a given genotype.
- The norm of reaction is a way to measure the change in phenotypes, while plasticity is the actual act of changing the phenotype to better suit the environment
- For a particular phenotype, the steeper the slope indicates a greater norm of reaction.
- X → environmental gradient
- Y→ phenotype
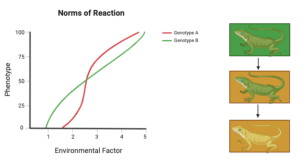
Key Takeaways
Overarching Themes and Unifying Concepts:
- Natural selection is the only evolutionary process that increases fitness and results in adaptation to environmental conditions.
- Variation is required for natural selection to occur. The variation must be genetic and heritable, and natural selection must lead to differences in survival and reproduction.
- Variation arises through a combination of genetic mutations, environmental influences, and the interplay of genes and environment. Variations within populations allow species to adapt to changing environments over time, ensuring the continued survival and success of different species in their respective ecosystems.
- Fitness is directly related to an individual’s ability to survive in its environment until able to successfully reproduce.
- Adaptation is context-dependent; an adaptation beneficial in one context could be detrimental in another context.
- Morphological and physiological adaptation is necessary for the survival of a species.
- The gut microbiome provides an additional “layer” of adaptation, by helping digest the diet.
- Humans have the capacity to drive evolutionary change.
- Populations that are flexible enough to adapt to anthropogenic pressures may have an advantage when adapting to other environmental changes.
- Species that are already challenged by anthropogenic pressures may be less able to respond to additional pressures.
- The benefits associated with adaptation often involve trade-offs.
How might this information be applied to address grand challenges?
- Evolution is especially important in the development of resistance to antibiotics, insecticides, etc.
- For better management and conservation techniques, it is crucial to understand how populations will adapt to changing conditions (or their lack of adaptability in changing environments).
- We could use modeling to anticipate future conditions and evolutionary changes in populations, and identify populations that will become at-risk in the future.
- If we are able to anticipate how humans’ behaviors will drive evolutionary change, we can promote measures to offset the change or, if possible, guard against causing deleterious selective pressures.
Unsolved Mysteries and Future Research:
- How do we determine if a trait is adaptive as opposed to a non-damaging trait that simply did not get selected against?
- How does coevolution influence microbial changes?
- As far as evolution goes, how did things such as sex, language, and even life in general originate?
- What would be the difference in phenotype expression- and implications of evolution- of small changes in DNA over time versus one large DNA mutation event?
- A comparison of animal and plant adaptations to certain ecosystems would be very interesting and useful to understanding interactions between all organisms and their surroundings in an ecosystem. Adaptations go a long way in describing these interactions.
Blog Style Summary
Have you ever wondered what it’s like to take AEC 400 with Dr. McKenney? If so, we have all the tea on the class. From Tuesday lectures to BioRender drawings and Hypothesis annotations, the class comes together in a unique and interactive way, just like a jigsaw. Applied ecology dives into the relationship between the environment around us and the human responsibility to take care of it.
You may have heard of Charles Darwin and his finches, but natural selection is only one aspect of ecology that represents species changing over time. Natural selection comes from a response to environmental changes, which increases fitness for some individuals in a population. Individuals with high fitness in this context get to contribute their DNA to the next generation, and over many generations, we would see patterns shift to traits favorable for survival. Natural selection is the only evolutionary pattern that results in adaptation. All species must adapt to some extent to their environment. Genetic variation of heritable traits is required for natural selection to occur.
Natural selection of certain traits can over time lead to either directional, stabilizing, or disruptive selection (Figure 4). Stabilizing selection narrows the variability of traits in a population. Disruptive selection is when traits on both ends of the spectrum are increased and middle ground is decreased, while directional stabilization pushes traits toward either side of the spectrum. Although this process can occur through natural selection, it can also occur through “unnatural selection”. As seen in a scientific study about the harvesting of wild animals, when humans hunt animals with larger weapons and body size, the population decreases to suboptimal phenotypes (Allendorf, F. W., & Hard, J. J., 2009). This change affects gene frequency in future generations and their ability to grow to larger sizes. Other impacts that alter genetic variation are mutation, genetic drift, reproduction, migration, gene flow, and assortative mating. When a change in phenotypes is observed, ecologists strive to determine if it is the result of genetic changes or just their environment.
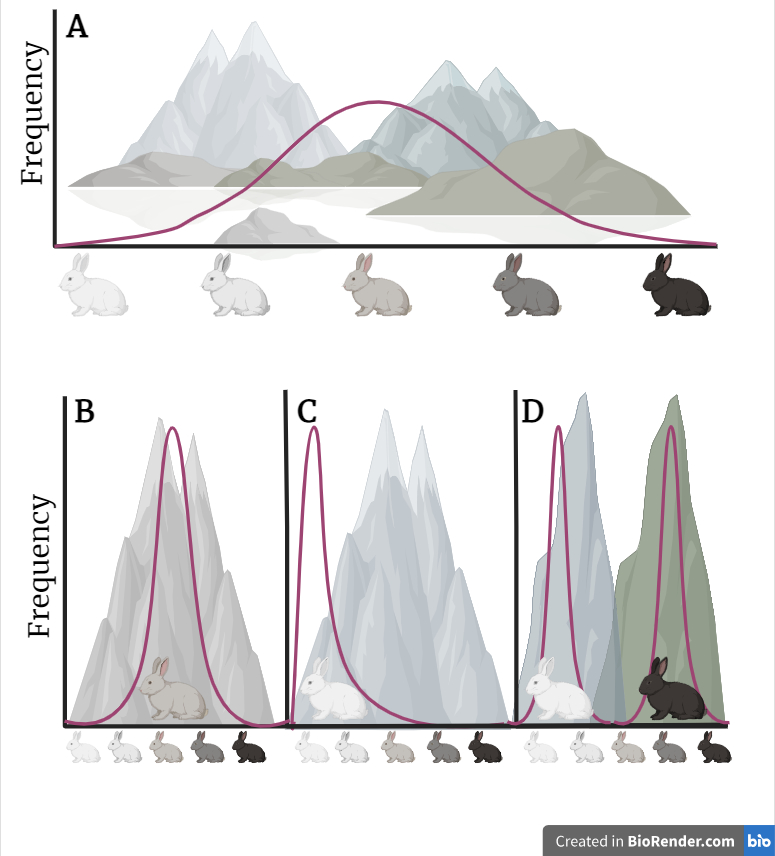
In order to determine if a change in phenotypes, which are physical changes, versus a change in genotypes, which are sets of alleles in DNA that influence phenotype, has occurred, there are experiments that can be done. The reciprocal transplant experiment and common garden experiment are used to determine the influences of environment and genotypes on phenotypes, though the method of determining it differs. In a reciprocal transplant experiment, individuals of the same species from 2 or more different environments are introduced into the other environment(s). If the individuals are able to shift their phenotype to match their environment, the phenotype is plastic and controlled by environmental factors; if the phenotype of both remains the same it is determined by genetics only. In a common garden experiment, ecologists study different populations reared under the same conditions to separate genotype and environmental influences on phenotype. If a population gives rise to offspring with the same phenotype in the novel environment, the trait is primarily influenced by the environment and therefore results from phenotypic plasticity. If the offspring express different phenotypes, the trait is controlled primarily by genotype. Phenotypic plasticity is when a single genotype gives rise to different phenotypes based on environmental conditions. A scientific study of coral microbiomes revealed that the corals on a given reef all “share a common gene pool”, and that the functions of specific genes in each microbiome correlated to the reef’s geographic location (Kelly et al. 2014). This also represents the norm of reaction where there is a range of phenotypic variation from the same genotype across differing environmental conditions. This broad range of genes allows coral to acclimate based on the temperature. Acclimation is when organisms are able to adjust to changes in their environment, in this instance, temperature. The researchers found that the corals had the highest nutrient composition when located near the equator, and nutrients decreased as latitude increased. Therefore higher temperatures were a selective force that acted on the phenotypes causing the coral to acclimate to its environment (Kelly et al. 2014).
Now the great thing about acclimation is its reversibility, so if a species needs to alter its phenotype in order to survive it will be able to; however, not all adaptations are reversible. In developmental plasticity, the changes that occur are irreversible: once an animal has adapted there is no way to change back. This can be seen with insects; many insects are a certain color based on the climate of their environment, with warmer climates favoring lighter colors and cooler climates favoring darker colors. One European study looked at the distribution of dragonflies from 1988 to 2006 to see the effects of global warming on coloration. Their research found that rising temperatures were allowing lighter-colored dragonflies to expand their range and they expected darker-colored dragonflies to shift their distribution to other areas, as well (Zeuss et al. 2014).
Now that we’ve gone over what natural selection looks like generally, let’s take a look at a specific example to differentiate between genotype and phenotype. Say you have a red bird with the alleles AA. Here, the bird’s genotype would be AA, and the physical representation, or the phenotype, would be red. If this red bird with genotype AA mates with a white bird with genotype aa, the following Punnett Square will be formed:
A | A | |
a | Aa | Aa |
a | Aa | Aa |
Using this Punnett square, we see that all the baby birds have genotype Aa and are heterozygous, meaning that they have two different alleles in their genotype. But, their phenotype is still red, since the allele that codes for red is dominant, which means that it is always represented in the phenotype.
Let’s see what a cross between two birds with genotype Aa would look like:
A | a | |
A | AA | Aa |
a | Aa | aa |
In this Punnett square, we see that a quarter of the birds will have the AA genotype which means that their phenotype will be red. Half will have Aa, and since the red allele is dominant, these birds will also have a red phenotype. The last quarter of the birds will have genotype aa, and they will be white, since their recessive alleles can only be expressed in the absence of a dominant allele. Keep in mind that half of the birds will be heterozygous with genotype Aa, while the other half will be homozygous, meaning having the same alleles, with either genotype AA (homozygous dominant) or genotype aa (homozygous recessive).
While non-human animals have been seen to evolve throughout different generations, the same concept also applies to humans. Our ability to drink milk as adults is an evolutionary feat. Selective pressures like immunity to disease and famine are thought to have favored a gene mutation that allowed adults to retain their ability to drink milk. These lactose-tolerant people would have had a better chance at surviving the disease and famine present throughout most of Northern Europe and East Africa at that time (Thompson 2011). Therefore, the lactose-tolerant gene was selected for in future generations, creating a directional change to favor the ability to digest milk as adults.
As time continues, we’re sure to experience much more evolution, both within ourselves and in the world around us. Understanding these ecological concepts helps with ecosystem management and conservation. We can work towards anticipating the future conditions and evolutionary patterns that may occur for a population of species, and act accordingly to protect species from extinction. From a human perspective, it is also helpful to understand evolutionary biology to understand the mechanisms of antibiotic and pesticide resistance in medicine and agriculture.
Spotlight on NC
A shared experience that many people who grew up attending school in North Carolina have is learning about the three geographical regions that make up the state. These regions are known as the Mountains, the Piedmont, and the Coastal Plains and each have very distinctive characteristics. The Mountains in the west have a relatively higher elevation with hilly terrain and forests. The central Piedmont region has gentle hills with both field and forest ecosystems. Lastly in the east, the Coastal Plains are characterized by low, flat land with many marsh-like areas. With such diversity in environments across the state, many species of organisms are able to flourish. Species in North Carolina have evolved and adapted in a variety of ways to live in these different regions of the state. This post examines an organism that can be found in each of the three regions in North Carolina, and how adaptation and evolution has helped them to thrive in these varying environments.
With its cool forests and many freshwater rivers and streams, the Southern Appalachian Mountains harbor 77 of the world’s known 550 salamander species, and are sometimes referred to as “The Salamander Capital of the World” [1]. With announcements of new species fairly regular in the news, have you ever wondered how new species occur? Just like Darwin and his different species of finches that arose from living on different islands, Appalachian salamanders diversify from inhabiting isolated “islands in the sky” in the form of mountaintops. Subpopulations of salamanders would have arisen from an original population as groups took up residence in different locales. Speciation because of a geographic barrier, like mountains in this case, is an example of allopatric speciation. If subpopulations of salamanders remain isolated long enough, they can eventually become a subspecies and finally their own species from their parent group, in a process driven by natural selection called species radiation. Some examples of features that denote different salamander species are coloration and tail length, which could arise from variation in diet, predators, humidity, or any other selection pressures unique to the salamander’s mountaintop. Salamanders have been around for 200 million years [2], and the amphibians have undergone substantial changes during that time to evade mass extinctions and stay fit for their environments. The Appalachian mountain range in and around North Carolina has provided salamanders with a uniquely well-suited environment, but with the climate changing at a faster rate than before, salamanders will be challenged to adapt at a rapid pace to avoid yet another possible mass extinction.
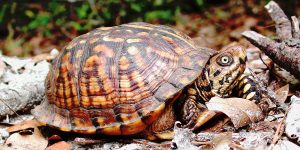
While eastern box turtles (Terrapene carolina carolina) can be found in much of the eastern United States, they are often associated with the Piedmont region of North Carolina [4]. These reptiles can be found in a variety of places such as fields, forests, and suburban neighborhoods. One adaptation that eastern box turtles have that allow them to survive in this range of places is their strong, highly-domed shells. Through natural selection, most eastern box turtles have especially hard shells that they can hide in to help protect them from predators or other threats. Natural selection can be seen in this example as turtles with stronger, tougher shells were able to survive better than turtles with weaker shells, thus being the individuals to reproduce more frequently. Another adaptation that relates to the shells of box turtles are the colorful markings that are often found on their shells. The phenotype for shapes and colors on their shells can vary from streaks to blotches and yellow to red [5]. These variations in color and shape help the turtles camouflage in their environment against the leaves and debris that can be found on the ground of the Piedmont region. The camouflage increases their fitness (i.e., survival and reproduction, to ultimately contribute their genes to the next generation) as they are better able to hide from predators and remain undetected. An adaptation that aids in their own hunt for food is eyes that are directed forward. Over many generations, more turtles that have forward-facing eyes have survived and reproduced as they have an easier time tracking prey and seeing other food sources. Even though eastern box turtles are not considered a keystone species, they are still important in many ecosystems as they inhabit such a wide range of the United States. Some of their roles in ecosystems include dispersing seeds, creating and modifying habitats for other species, and impacting nutrient cycling [7].
One aquatic reptile native to the North Carolina coast is the Loggerhead sea turtle (Caretta caretta), the most common species of sea turtle in NC. The Loggerhead plays a key role in both the environment and ecotourism for the coastal plains region. Females bury their eggs in the sandy dunes along the beaches. North Carolinians play a role in protecting these turtles by protecting the eggs and hatchlings. This support is essential to their survival since they are globally classified as “Vulnerable” to extinction by the IUCN Red List, and “Threatened” with extinction at both the federal and state level (source- IUCN). Loggerheads also help NC attract tourists from across the world by eating species that could be considered a nuisance to tourists, such as crustaceans and jellyfish [8]. Loggerhead shells also provide a substrate that supports additional species that are able to colonize the surface habitat, further increasing ocean biodiversity! It is important to notice the adaptations that Loggerhead turtles have acquired in response to beach conditions on the coast of NC – or need to acquire due to environmental changes as North Carolina’s climate changes. Some of their adaptations to live in water include their flippers, which help them swim faster and more efficiently when compared to terrestrial turtles [8]. Another adaptation is that they are ectothermic, meaning that loggerheads and some other species of sea turtles don’t regulate their temperature by themselves: their body temperature is influenced by the temperature of their environment [9]. Because of their unique relationship with water temperature, warming oceans are causing a host of issues in these turtles from weakened immune systems to difficulties reproducing.
One unique thing about the Loggerhead sea turtle is that they travel all across the world to find a mate. However, we are now seeing changes in turtle breeding behaviors due to acclimation as well as phenotypic plasticity. A study showed that sea turtles are migrating away from the equator and towards the poles to breed because of rising temperatures. This temperature-dependent behavior is reversible, and thus an example of acclimation. Rising temperatures are also causing earlier nesting seasons, which can contribute to the change in sex ratios of hatchlings, which is temperature dependent [10]. Because turtle hatchlings’ sex is an irreversible trait, the change in sex ratios is an example of phenotypic plasticity. This is problematic because sea turtle populations may not be able to adapt or tolerate the increasing temperatures resulting from climate change. Continued migration may result in overcrowded nesting grounds, negatively affecting the fitness of adult females who come to lay eggs on North Carolina’s beaches as well as survival rates of resulting hatchlings. And, as temperatures continue to climb, future generations will experience increasingly female-skewed sex ratios. With so few Loggerheads left, analyzing how climate change is affecting breeding patterns is essential to their survival, as well as the conservation of the coastal North Carolina ecosystem as a whole.
The gradient of habitats across North Carolina has certainly given rise to some amazing wildlife, with our three case studies highlighting prime examples. Not many states can boast mountains, rolling green hills, and a coastline, but all of this diversity also means that more species are at risk of extinction due to the ongoing climate crisis. Salamanders and turtles, both aquatic and terrestrial, have enriched humanity even as our extractive tendencies have harmed them. We are ultimately waiting to see if these and other species will adapt to human impacts, but it may be too late for many species by the time those impacts are observed. In the meantime, we can try to make changes to keep our beautiful NC biodiversity alive. Appreciating the millions of years of natural selection, adaptations, and ultimately speciation that have culminated in the animals that we get to interact with today is an important part of properly caring about and for these animals. By modifying our habits, as individuals and as our own species, we can hope to decrease our effects on these species with whom we share the planet.
How to Apply Ecology in a Changing World
Humans quickly change ecosystems and the environmental factors influencing many species. Conservationists must consider how we should adjust our own lives and infrastructures to avoid a dramatic decrease of wildlife populations. Hunting affects animal populations by decreasing their population size, and usually they are able to recover with relative ease. However, selective hunting for specific phenotypes can rapidly change a population’s gene pool. For example, trophy hunters in the US tend to shoot for buck with large antlers. This can cause a rapid decrease in bucks with large antlers, a phenotype that is preferred in assortative mating [1]. This change in the gene pool causes the population to experience artificial selection as humans are removing the most impressive individuals from the population. This can erase the effects of thousands of years of evolution, and many species cannot recover from such strong anthropogenic pressure. While artificial selection can be detrimental for any species, it is even worse for species that are already struggling with habitat loss, and often contributes to their classification as endangered or threatened species.
Historically, colonization has led to the mismanagement and extinction of species around the world. The red wolf (Canis rufus) is an excellent and well-known example of extinction due to mismanagement. Once known to range across the southeastern United States, the red wolf is now considered extinct in the wild. A small number were recovered in Texas and brought to wildlife refuges in coastal North Carolina for reintroduction [2]. Zoo nutritionists and other wildlife caretakers must use clues from an animal’s physical characteristics to determine which foods and habitat features will satisfy each species’ niche-specific requirements. Biologists and conservationists must use ecology in a similar way to determine where endangered species would thrive best after they are relocated.
Conservationists have discussed translocating endangered species into new habitats for decades, but not many trials have moved species outside of their historic range [3]. Recently, Guam rails have been introduced to the island of Rota, even though that island has never been a habitat for the flightless birds [4]. Sometimes called “assisted migration,” the goal is to move endangered species to a habitat that matches the niche they evolved for – without creating an invasive species or damaging the ecosystem [4]. This practice would be most relevant to wild animals endemic to islands facing habitat loss and for plant species that cannot migrate quickly to more hospitable areas [3][5]. In the case of the Guam rails, their home island has become completely uninhabitable after the introduction of a new predator, the brown tree snake [4]. The snake has become a threat to other Guam inhabitants; the Guam kingfisher (or sihek) may also require an assisted migration to increase the species’ chance of survival in the wild [6]. Ongoing monitoring of assisted migration programs such as the efforts on behalf of Torreya taxifolia (Figure 6) will show whether it is a useful tool to support endangered species, or if it creates more problems than it solves.
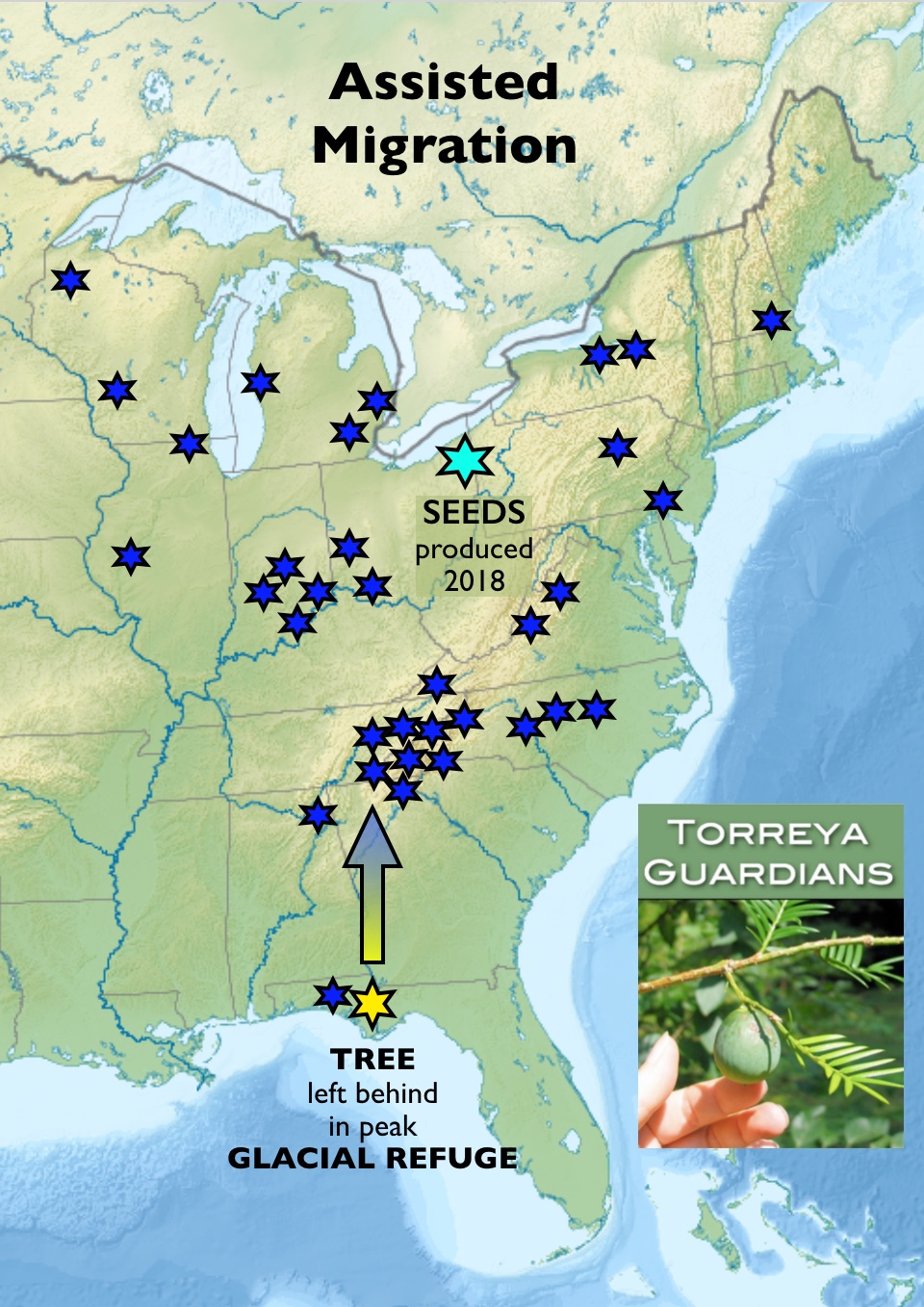
Featured Ecologists:
Dr. Patricia Bedinger, PhD
Dr. Patricia Bedinger studies the reproductive barriers of wild plant species, especially wild tomato species. In her lab, she researches the molecular and cellular nature of inter-specific reproductive barriers (IRB). Dr. Bedinger has done extensive research on the molecular basis of these reproductive barriers between about a dozen wild tomato species from Latin America and has identified both pollen and pistil factors that are involved in post-pollination barriers. Dr. Bedinger now wants to determine the role of these barriers in the evolution of any new lineages of wild tomatoes by studying incipient barriers. Additionally, Dr. Bedinger also studies in her lab the regulation of pollen tube growth and development and the role of extensins in the walls of plant cells. She uses a variety of resources to accomplish these studies such as genetic analysis, genome sequencing, and hybridizing different wild plant species. While reading her work and journal articles, I found similarities in her work to our class, such as genetic differentiation, life history traits, and the role of inter- and intra-specific competition in a species’ reproduction. Her research increases scientific knowledge on the role of IRB in wild plant species that are of agricultural importance. Understanding these factors has implications for the fields of horticulture, agroecology, and sustainability.
Dr. Sharlene E. Santana, PhD
Dr. Sharlene Santana explores adaptive radiation in bats by examining their morphology and ecological relationships. Some of the main ecological concepts used in her research that we have examined in class include adaptive radiation/niche specialization, mutualism, ecosystem ecology, and coevolution. Examples of her major findings include: describing evolutionary pressures select for specific skull shapes in bats (diet and echolocation), describing the complex organization of the neotropical ecosystem where frugivorous and nectarivorous bats reside, and using novel DiceCT technology to describe the masticatory musculature of a wide array of bats. Her research has given many insights into the evolutionary history of bats along with their roles in ecosystems today, and while her career is still very much upcoming rather than behind her, her studies will hopefully inspire others to conduct similar research on other taxonomic groups.
Pamela Yeh studies the effects of human impacts and novel environments on evolution in birds and bacteria. In the lab, Dr. Yeh studies drug resistant bacteria and the reaction of bacteria to antibiotics. In the field, Dr. Yeh studies the impact of urbanization on birds, specifically dark eyed juncos and house sparrows. While this research is still ongoing, Dr. Yeh is studying the effects of multiple stressors on microbes and how they affect selection and adaptation. They are developing strategies to prevent the evolution of bacteria that are resistant to multiple drugs, and hope to use this research to solve public health issues. Dr. Yeh’s studies on birds are also still ongoing, but their results can tell us more about the adaptation of organisms to urban environments.
Student contributors:
Note Outline: Cassidy Davis, Vanesa Gutierrez, Lauren Pfau, Sophia Uthe, Emma Wilson
Blog Style Summary: Elissa Cunane, Em Trentham, Saniya Pangare, Alana Brown
Spotlight on NC: Claire Sholar, Christina Conrad, Alex Trifaro
Featured Ecologists: Rachel Weaver, Cassidy E. Davis, Marley Kelly
How to Apply Ecology in a Changing World: Paige Mesecar, Kaya Rosselle, Andrea Aguirre Salazar
a species with a small body size and short life span that produces lots of offspring earlier in life. Offspring have a low survival rate and low level of parental investment
plural of locus, the actual fixed positions of the gene within the chromosome
the mechanism of evolution; environmental pressures result in differential survival and reproduction rates, causing different phenotypes (and adaptations) to become more prevalent in a population
Requires variation and heritability that leads to differences in fitness
morphological, behavioral, and physiological traits that increase fitness in a particular environment
physical manifestation of DNA
set of alleles in DNA that influence phenotype
different form of a gene
swap the conditions under which two different ecotypes are reared
Determines genetic component to phenotype
Rear offspring with different genotypes under same environmental condition
Determines genetic component to phenotype
single genotype gives rise to different phenotypes based on environmental conditions
Phenotypic plasticity in itself is a heritable trait (plasticity with respect to a given trait)
process in which an individual organism adjusts to a change in its environment (reversible process)
a similar process to acclimation, except it is permanent
two different alleles for a gene
two of the same alleles for a gene