Chapter 10: Food Webs
Vocabulary:
- Apex Predator: carnivore at the top of a food chain with no natural predators. Commonly keystone species.
- Apparent competition: prey species share a predator, but do not compete for resources. The competition is only “apparent” because of the surrounding interactions.
- Basal Species: species with no prey in a food web and commonly shown at the bottom. These are usually autotrophs or detritivores.
- autotroph: an organism that produces its own energy form sunlight (photosynthesis; e.g., any green plant) or chemical reactions (chemosynthesis; e.g., deep sea bacteria)
- detritivore: an organism that consumes dead and/or decaying organic matter (detritus)
- Bottom-up control: the productivity and abundance of populations at any given trophic level are limited by the productivity and abundance of populations below them.
- Connectance: the proportion of possible links between species that are realized, calculated as the number of links divided by the maximum number of possible links
- Linkage density: the average number of links per species; measures the complexity of interactions across a food web
- Top-down control: predator populations limit the abundance of prey species, often resulting in a trophic cascade.
- Trophic cascade: occurs when top predator populations change, causing reciprocal changes in the populations of lower trophic levels. Often results in drastic changes to ecosystem structure and functioning.
- Trophic position: shows where certain species fit in relation to all other species in that trophic level.
- Calculated from the fraction of food they consume from other trophic positions [1]
Outline of Notes:
Food Webs:
- Food webs are a way of visualizing relationships between organisms based on their trophic level.
- An organism’s trophic level is determined by its feeding behavior:
- Top/Apex predators occupy the highest trophic level
- Intermediate species occupy the trophic levels between top predators and basal species:
- Mesopredators sit just below top predators
- Herbivores sit above autotrophs
- Basal species occupy the lowest trophic level
- Usually autotrophs or detritivores (decomposers)
- An organism’s trophic level is determined by its feeding behavior:
- Arrows are used to indicate the type of interaction occurring between organisms:
- Up arrows (↑): Indicate the flow of energy from food item to consumer
- Down arrows (↓): Indicate regulatory effects from one population to another
- These can be positive or negative, and indirect or direct
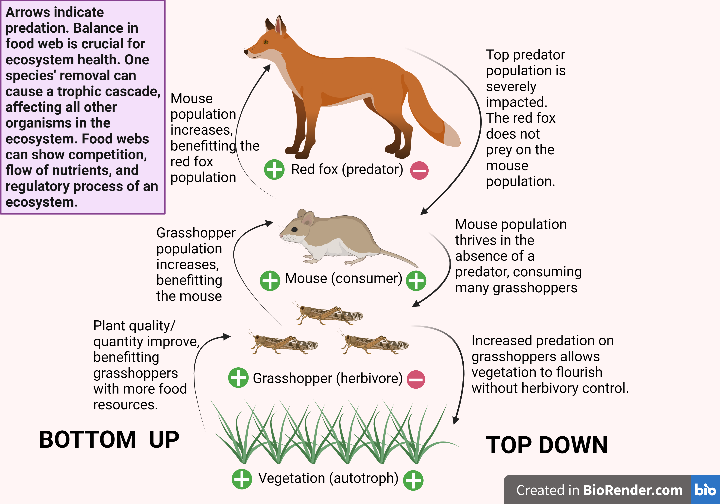
-
- Example: Predators have a direct negative effect on herbivores, and an indirect positive effect on plants. Predators directly reduce herbivore populations by consuming them, thus reducing grazing on plant populations, allowing plant populations to increase.
-
Figure 2. In this simplified food web, P = top predator, C = Consumer, H = herbivore, and A = autotroph. Note that consumers include both carnivores (just not the top predator) and omnivores (which consume both plant and animal tissues). Food webs are organized vertically by trophic level, with top predators at the top of the food web and basal species at the bottom. What can food webs tell us about a species?
- Feeding strategies
- Generalist vs specialist
- Competition
- Possible trophic cascades
Quantifying Food Web Dynamics
- S = number of species
- L = number of links (arrows)
- S2 = maximum number of links
- C = Connectance = L/S2
- Connectance: the proportion of possible links between species that are realized
- LD = linkage density = L/S
- Linkage density: average number of links per species. Measures the complexity of interactions across a food web.
- Chain length: average the number of arrows from an autotroph to an apex predator.

- Mean chain length (ChLen) = the average length of all observed chains in the food web.
1. Calculate the length of every chain (= total length)
2. Divide the total length by the total number of chains in the food length

- Compartmentalization:
- Food web structure and species interactions become more complex as species richness increases.
- Body size, prey selection, and habitat use may all contribute to compartmentalization.
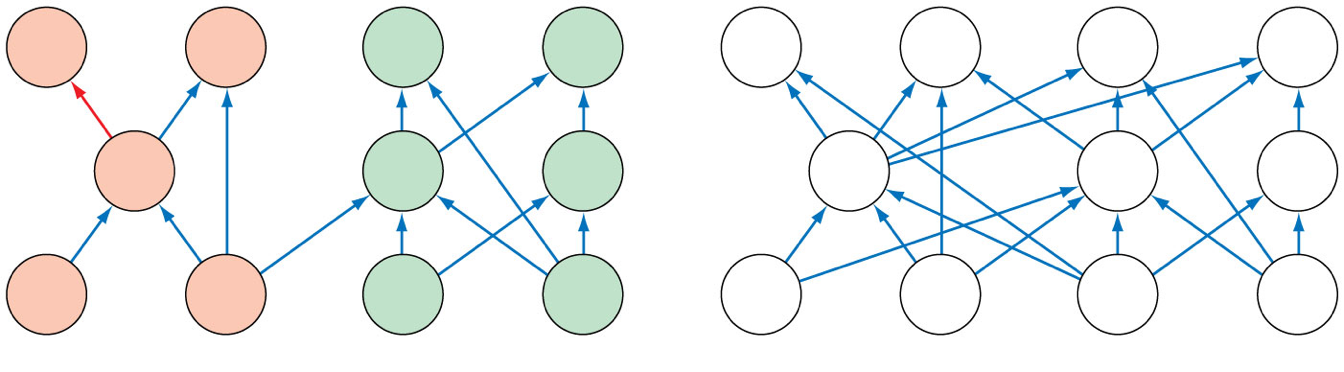

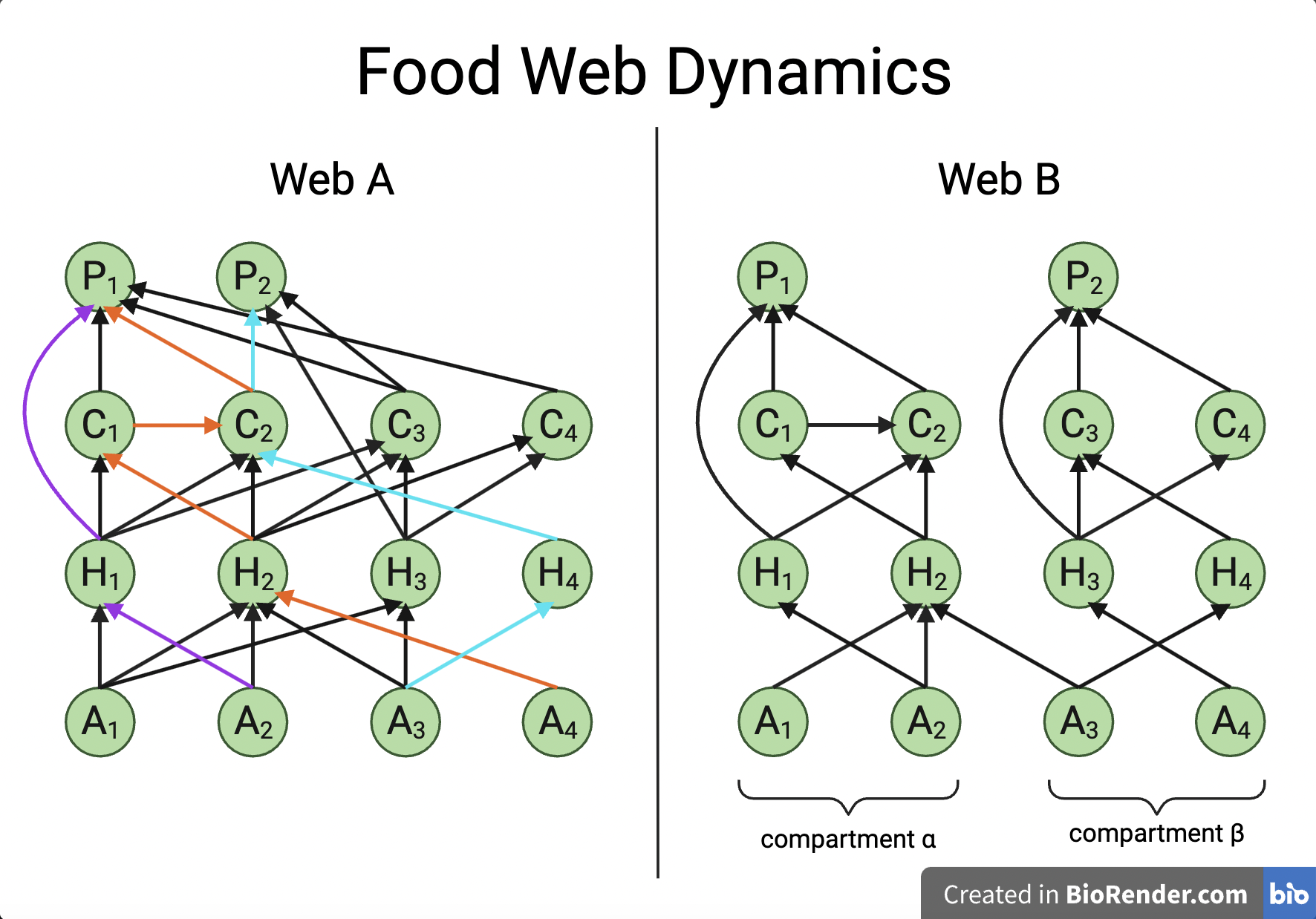
Food web feature |
Why do these features matter? (What do they represent?) |
Number of species (S) |
Biodiversity |
Number of links (L) |
Species interactions and keystone species |
Connectance, linkage density (C, LD) |
Co-evolution and co-dependence among species |
Chain length |
Identify umbrella species and the potential strength of a trophic cascade |
Compartmentalization |
Identify forces that drive inter-species interactions, and/or separate members of a community |
Species Interactions:
Food webs can illustrate different species interactions.
- Species interactions can be direct or diffuse:
- Direct relationship: a one-to-one relationship between two species.
- Ex: A specific pollinator may only visit a specific flower, and that flower may only be visited by that one pollinator species.
- Diffuse relationship: one species may interact with several other species.
- Ex: A pollinator, like a bee, may visit several different types of flowers
- Ex: A flower may be visited by many different species of pollinators
- Direct relationship: a one-to-one relationship between two species.
- Species interactions can also be direct or indirect:
- Direct interactions: occur when species interact through direct contact.
- Ex: predation
- Indirect interactions: species interact through changes in another species
- Ex: an increase in lion populations may cause an increase in grass abundance by decreasing antelope populations.
- Apparent competition: two species do not compete for resources, but they do share a common predator.
- An increase in one prey population will increase the predator’s population, which increases predation pressure (i.e., consumption) of the other species as well – making it appear that the two species are competing, because one population decreases after the other increases.
- Ex: An increase in the population of kudu (H1) increases the populations of lions (P). An increase in lion populations increases predation pressure on both kudu (H1) and zebras (H2), ultimately decreasing zebra populations (H2).
- The increase in kudu abundance (H1) corresponding to the decrease in zebra abundance (H2) appears to be a competitive interaction, even though the two species do not directly compete for resources. (Kudu are browsers, while zebra are grazers.)
- Direct interactions: occur when species interact through direct contact.
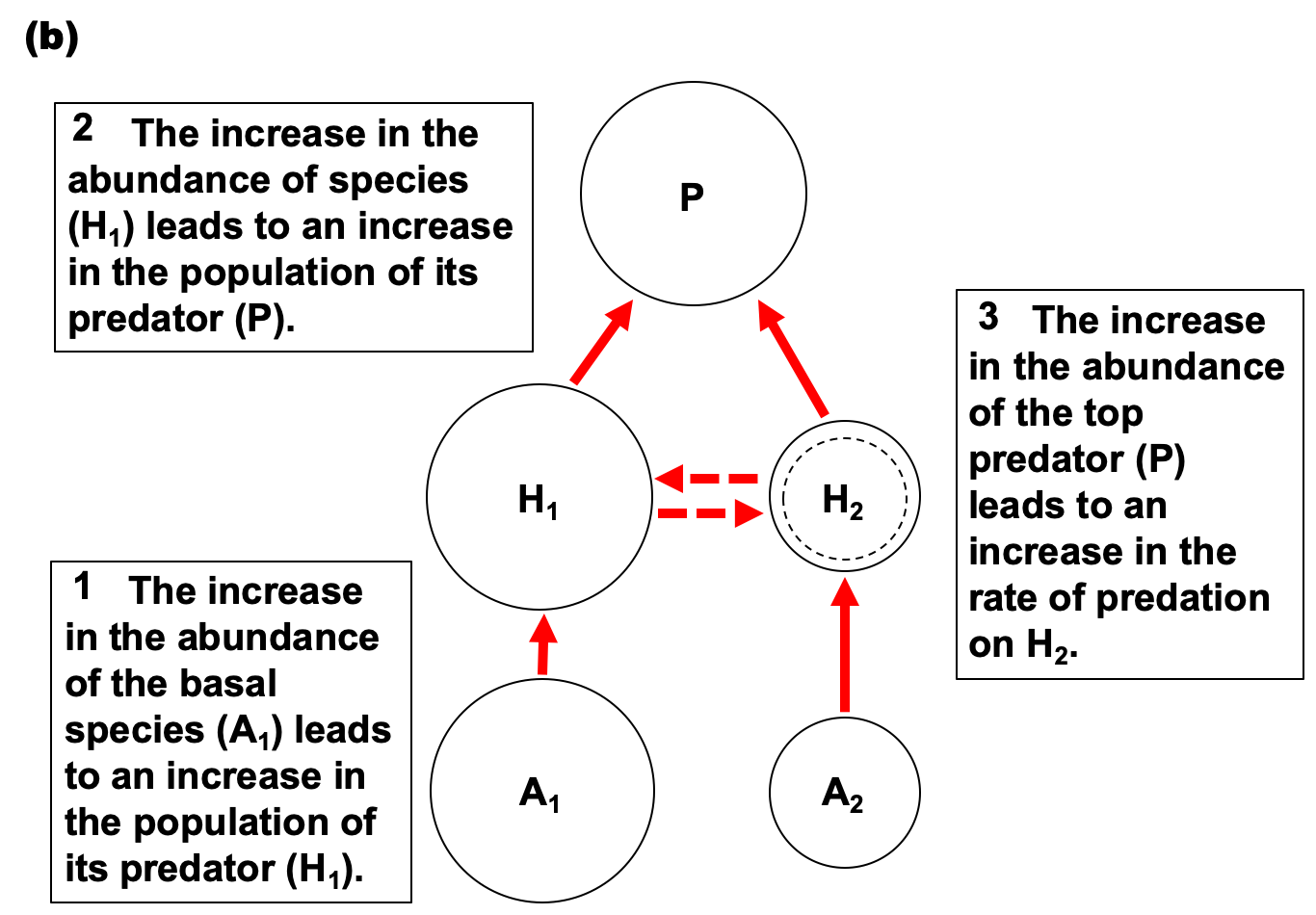
Question from a Student:
“Does apparent competition imply that the species that are preyed upon have a similar niche or ecological role?”
Answer, from Andrew Joravic and Jordan Holcomb: Apparent competition does not necessarily imply that the species have similar ecological niches or roles. The key factor in apparent competition is the shared predator, not the similarity of the preys’ ecological niches. This concept doesn’t have much to do with the prey niche spaces, because no two species can occupy the same ecological niche for long.
Regulatory Effects on Food Webs:
- Top-down control: predator populations limit the abundance of prey species, often resulting in a trophic cascade.
- Trophic cascade: occurs when top predator populations change, causing reciprocal changes in the populations of lower trophic levels. Often results in drastic changes to ecosystem structure and functioning.
- Bottom-up control: the productivity and abundance of populations at any given trophic level are limited by the productivity and abundance of populations below them.
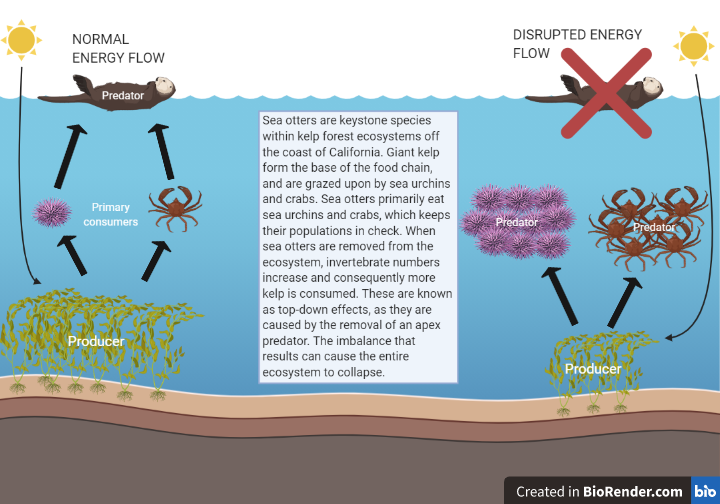
Question from a Student:
“What are some of the distinctions between bottom-up control and habitat management? I understand the latter can involve abiotic factors, but those can be difficult to “manage” in an adjustable way – changing salinity or the mineral composition of a landscape is very difficult, and adjusting the availability of water and soil structure is also very difficult without disrupting the ecosystem as a whole. Is habitat or landscape management synonymous otherwise, or is bottom-up control something that solely focuses on an individual factor towards ‘the bottom” of the food web? What is an example anyone can think of?”
Answer from Sarah Alanbagi: Bottom up control mainly focuses on the roles of primary producers (basal species) in a given ecosystem. (Do they have enough nutrients? Are they facing extreme herbivory?) Habitat management includes bottom up as well as top down control, stream management, fire ecology, etc.
Key Takeaways
Overarching Themes and Unifying Concepts:
- Food webs are very complex – and that complexity can be quantified!
- A change in one trophic level can affect species in other trophic levels as well as others in that trophic level
- Important information about community and ecosystem ecology can be inferred using food webs such as predator-prey relations, competition between species, the flow of energy and nutrients, and regulatory effects.
How might this information be applied to address grand challenges?
- Food webs help us protect endangered species, as they allow us to understand which species support each other and which might need to be managed.
Unsolved Mysteries and Future Research:
- There is a lot of uncertainty in how exotic species affect food webs, for example, the inconsistent interactions between lake trout and invasive lampreys in the Great Lakes. Future studies that investigate succession after the introduction of invasives could help remedy this by revealing larger-scale interactions of invasive introductions, not just a handful of direct ones.
Blog-style Summary:
Food chains can be a very effective tool for wildlife conservation and management. Food chains typically show the main energy flow from primary producers (autotrophs), through consumers, to the apex predator. Food chains can be used to see what the effects may be of top-down or bottom-up control. Top-down control involves controlling predator populations in an effort to control the abundance of intermediate species, or prey populations; this type of control often results in a trophic cascade. Trophic cascades occur when top predators are added or removed, influencing the abundance of every organism that comprises the lower trophic levels of an ecosystem. Because food chains are incredibly simplified, they are best applied when looking at one specific species, such as wolves.
For example, if wolf populations are increased in an area, deer populations will decrease, allowing grass populations to increase as a result. Bottom-up control is the opposite approach to top-down; instead of changing the abundance of a top predator, the abundance and productivity of producers is modified. In our wolf example, if we increase the number of primary producers (grass), the amount of deer will increase, thus increasing the abundance of wolves in the ecosystem.
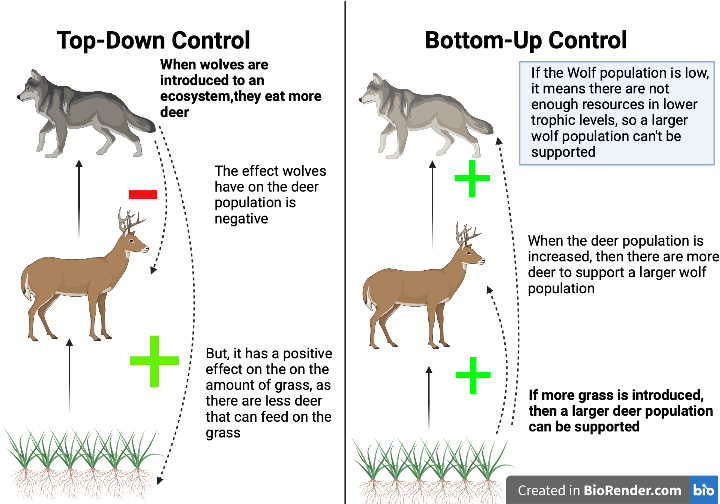
Food webs provide a more in-depth and accurate version of ecosystem interactions, by incorporating several individual food chains. While both webs and chains represent the flow of energy through an ecosystem, they serve different purposes. Most species consume multiple food sources and utilize a different food resource when their primary source is unavailable. In these instances, food webs are better as they include these alternate food sources. By visualizing the many species that interact and compete for available resources, food webs also enable ecologists to investigate and quantify relationships between species and across trophic levels.
For example, Trophic cascades are events that are initiated by either removing a top predator or introducing a top predator into an area. One of the best-known trophic cascades in the United States was documented in Yellowstone National Park. Following the removal of wolves in the 1920s, elk populations in the area were released from predation pressure and quickly grew. This growth in the elk population increased grazing pressure and caused plant populations in the area to decrease. In 1995 wolves were reintroduced back into Yellowstone National Park. Wolves began to prey on elk, causing a reduction in the elk population. This reduction in the elk population allowed the plant populations to recover and increase in abundance (Ripple et al. 2012).
Several types of interspecies interactions exist in a food web beyond predator and prey relationships. Competition, mutualism, predation, and parasitism all exist within food webs. When most people think of food webs they oversimplify it and assume that energy flows in a single direction toward the apex predator but in reality, it’s much more complicated. The direction and flow of nutrients in a system goes up through intermediate and apex predators, sideways between intermediates, and even cycles through decomposers to contribute to bottom-up effects. (Learn more about the detritus cycle in Chapter 11: Ecosystem Ecology.)
Food webs are an important tool for understanding the complex nature of relationships between every organism in an ecosystem. Understanding the complexity of these trophic interactions serves a multitude of purposes. When an invasive species is introduced into an ecosystem, the magnitude of the effects can be determined by how it changes the interactions in the food web. When we as a scientific community can understand these changes and anticipate future implications to the ecosystem, we can create a management plan that is tailored to the specific problem at hand.
Spotlight on NC:
Impacts of Feral Hogs in North Carolina
Background
Invasive feral swine have lived in North Carolina for centuries. Originally brought over from Europe for food and hunting, feral hogs today have become a nuisance causing crop destruction, forest destruction, and wetland damage [1]. This is largely caused by the generalist nature of the pigs. They have minimal predators to control their populations and will eat just about anything [1]. The species has become a nuisance for farmers by eating crops and trampling land. Their omnivorous diet allows them to wreak damage on a multitude of environments, eating things from insects to crops to forest understory [2]. Their rapid introduction has prevented the adaptation of native organisms to their behavior and diet [1]. Their invasive ways and generalist diet cause large impacts on the surrounding ecosystems’ food webs.
Food Web Applications
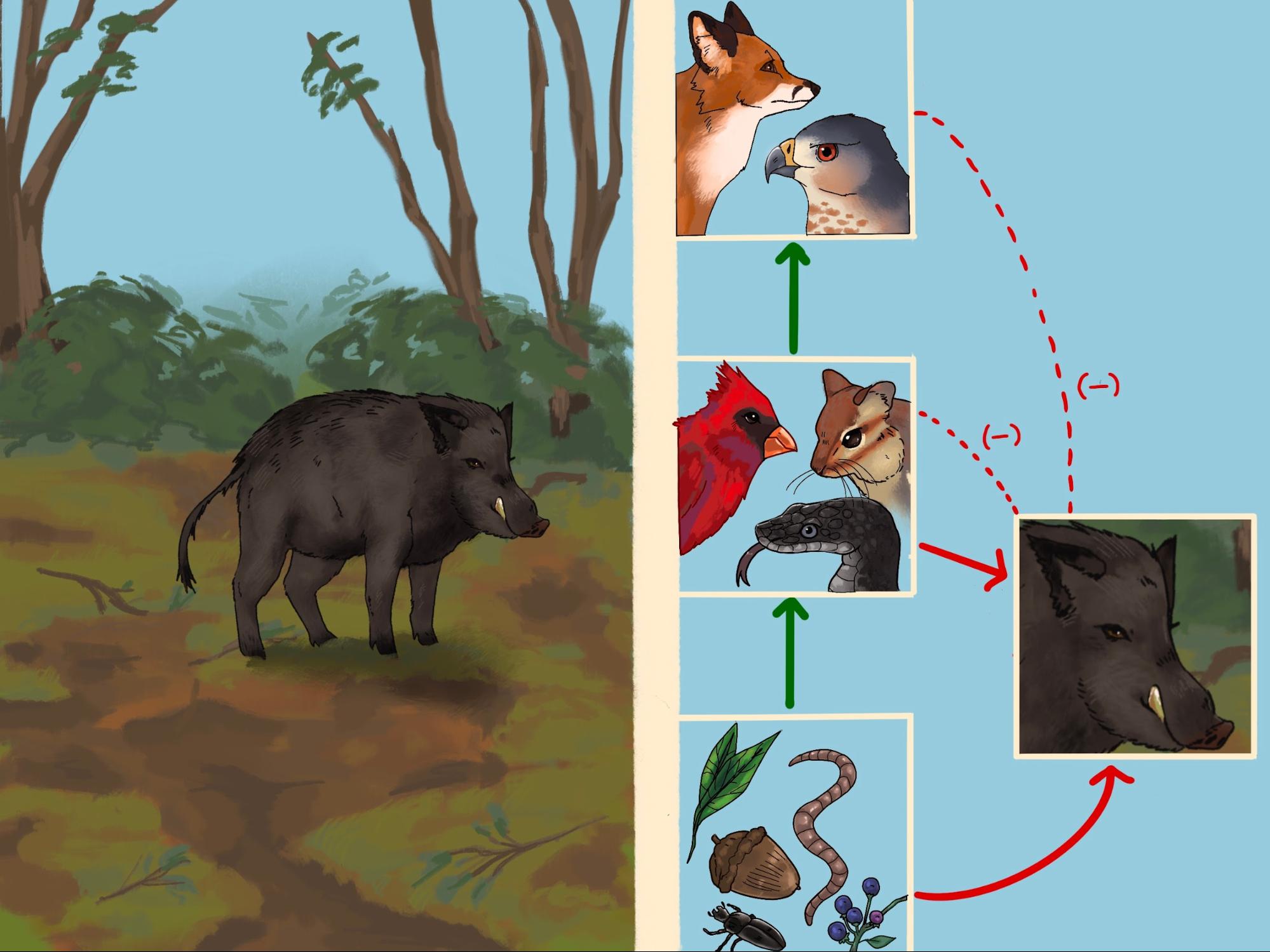
In North Carolina, feral hogs have spotty populations on the coast and in the Great Smoky Mountains. They are especially detrimental to mountain ecosystems since many sensitive plant and animal species reside there. [3]. They transform the environment through their foraging, turning up the soil as they search for plants and insects to eat. They can also cover large areas in a short amount of time and do not discriminate between natural and agricultural lands. While young hogs can be predated upon by coyotes, bobcats, and foxes, adults have no natural predators. This is due to a combination of their large adult size and rapid reproduction; any natural predators they may have are not able to keep the population in check [1]. This means that there are no top-down controls to their population. Since they are omnivorous generalists, their extremely broad diet prevents them from feeling the effects of
bottom-up controls as well.
Solutions and Proposed Research
When coming up with a solution for the issues created by feral hogs, one’s first thought might be to go hunting. Hunting is often used as a form of population control and is sometimes encouraged for certain invasive species and organisms [1]. However, this is not entirely the case for the feral hog. In areas where the hogs are already established, this practice could be an effective start to controlling the population. In states where feral hogs are not an established animal, though, it has become a popular practice for hunters to bring hogs en masse from other states to hunt for sport. This introduces the hogs to areas where they had not previously resided and furthers their invasive nature to states that did not previously have a hog issue [4].
Some of the methods of hog population control that have proved to be successful include aerial gunning, corral trapping, and poisoning [2]. In the practice of aerial gunning, aircrafts track these feral hogs and shoot them from above. Corral trapping can have lethal or nonlethal connotations depending on the area. However, in most areas, the lethal route is considered the most ethical and effective method, since the hogs cause so much destruction. Poisoning is another method of control that entails setting up bait sites and laying out poisoned foods in large amounts for the hogs to eat [1].
While these control methods have been heavily researched and tested, the level of ecological disturbances that the feral pig causes calls for more research to be done. It is our suggestion that further research goes into methods of lethal population control, including finding more effective methods of poisoning. This could perhaps even be done by finding ways to make the crops they destroy unappealing or poisonous to them, but not to humans. We also suggest more funding and research goes towards creating policies that ensure hunters do not continue this “catch-and-release” method of sport hunting.
Conclusion
Feral swine can majorly disrupt North Carolina’s ecosystem due to their invasive, omnivorous nature. If they remain, the hogs could spread and invade further areas [4]. Removing the hogs from these ecosystems could allow the food webs to return to normalcy, creating a healthier ecosystem [2].
How to Apply Ecology in a Changing World:
Human-Induced Trophic Cascades
Think about what you ate before opening up this textbook. Maybe you had a salad. Maybe you had a burger. Maybe you were really adventurous and had a protein bar made out of crickets (hey, we won’t judge). Now, think about what might eat you. Just kidding, don’t actually do that! Humans in industrialized / Westernized cultures tend to be apex predators, meaning that we’ve pretty much figured out how to avoid predation (though the Jaws and Jurassic Park movie franchises pretend otherwise). The vegetables making up your salad are basal species (specifically photoautotrophs – species that make their own energy using photosynthesis) that don’t prey on any other species. The cow making up the burger and the crickets in the protein bar are intermediate species, which both prey on and are preyed upon by other species. The links between basal species, intermediate species, and apex predators in this simple example form a food chain. In reality, these links would form what is known as a food web, where species are connected to many others through shared food sources (prey) and predators.
The inherent interconnectedness of food webs makes them sensitive to disturbance. Apex predators are particularly important in food webs of all ecosystems, whether in a meadow, a pond, a mountain, Narnia, or any other location you can think of. Changes in the abundance of the top predator species impact the prey species in the trophic level directly “below” them in the food web in different ways [1]. This can then have ripple effects that extend down to all other species in the food web, creating what is known as a trophic cascade. This is a form of top-down control in ecosystems, meaning that predator populations have a strong influence on prey populations (the opposite – bottom-up control – occurs when basal species limit the abundance of higher species on the food web). Trophic cascades can occur naturally, but humans just seem to love inducing them ourselves. Prominent examples of the detrimental impacts of human-induced trophic cascades are currently playing out in both marine and terrestrial ecosystems, representing a pressing challenge for our generation (and future ones) to solve.
Let’s use the Coral Triangle, a region in the Western Pacific Ocean, as a case study. The area is home to abundant fish species, coral reefs, and other marine organisms. However, the food web faces a significant threat from overfishing. Consider if the fish we catch is an intermediate species: if they are missing, the basal species will flourish, and the top predator will starve. If we overharvest an apex predator, the intermediate species will increase in abundance, and the basal species will decline due to overgrazing (this is the classic trophic cascade).

Overfishing occurs when the number of fish caught exceeds their ability to reproduce and maintain the population. In the Coral Triangle, this issue is worsened by various human activities, such as destructive fishing methods, inadequate fisheries management, and an increasing demand for seafood (both locally and internationally). The good news is that there are steps we can take to address overfishing in the Coral Triangle [2]:
- Establish regulations: Local governments within the Coral Triangle should set up regulations to control fishing activities, such as:
- Establishing a catch limit: minimum size fish that can be caught, and how many fish (amount/weight) one boat should not exceed per day.
- Determining the species of fish that should not be caught.
- Prohibiting destructive fishing methods (e.g. bottom trawling over vulnerable habitats).
- Instituting a no-catching zone: a zone where fishing is not allowed, which can help preserve biodiversity in a region [3].
- Enforce regulations: post fines for illegal fishing. Just like traffic tickets, some are more severe than others, and lead to different consequences.
- Encourage the use of selective and sustainable fishing gear to minimize bycatch and habitat damage: The government should support the promotion of sustainable gear, and reduce taxes on this gear.
- Education: Conduct educational campaigns to raise awareness among fishermen about the importance of sustainable fishing practices, the benefits of long-term conservation, and the long-term effect of overfishing.
Implementing these steps will require collaboration among governments, local communities, and private sectors. By having a better understanding of how the ecological system works, regulations can be set up, and people can better understand the whole picture of long-term health and sustainability of the marine ecosystem. If this happens, eventually, the Coral Triangle will overcome the challenge of overfishing and resulting trophic cascades.
Trophic cascades also occur in terrestrial ecosystems. Humans have been hunting for more than 1.8 million years; but over time (and especially as food became more accessible), the reasons people hunt have changed. With over 15.1 million hunters in the United States alone, overhunting has become a serious problem for many terrestrial ecosystems. Overhunting occurs when intermediate species or apex predators are killed at unsustainable levels, causing trophic cascades in the food web. Here are ways that overhunting occurs [4]:
- Hunting for food: deer, wild boar, etc.
- Hunting for trophy/sport: some people hunt for endangered animals to taxidermy them or hunt for bucks with large antlers.
- Hunting for economic gain: for example, people hunting elephants, walruses, or hippos to sell the ivory they harvest from them.
When people think about the problems overhunting can cause, they primarily think about what happens to the food web. However, various other ecological problems arise, such as [4]:
- Interrupting migration patterns: animals may change their path during migration if prey is no longer around.
- Affecting non-endangered animals: they might struggle to survive with low prey numbers.
- Affecting endangered animals: people sometimes target endangered animals when trophy hunting.
- Agricultural problems: Animals help keep insects/pests off of crops, but with diminishing numbers insects will thrive.
Fortunately, there are steps we can take to prevent overhunting (and thus trophic cascades) [4]:
- Stricter hunting laws: these would help protect animals and alert people on new or potential endangered species.
- More hunting laws: this could further protect animals by enforcing regulations on how much you can hunt, or what you can hunt.
- Stopping economic support on animal materials: for example, stopping the selling of ivory, or pangolin scales.
- More funds for conservation agencies: with more funding, conservation agencies could help spread the word of overhunting and the importance of protecting the animals living in our ecosystems.
Hunting does play an important role in the economy, by providing 27.1 billion dollars a year from equipment, traveling, and licenses. But the damages of overhunting far outweigh the money that it brings in. To help address overhunting we need help from government officials, the community, and people to spread the word about the problems hunting is causing. Through further educating people and bringing the problems to people with legal power, we believe we can solve the problems of overhunting.
References
- McPeek, M.A. (1998). THE CONSEQUENCES OF CHANGING THE TOP PREDATOR IN A FOOD WEB: A COMPARATIVE EXPERIMENTAL APPROACH. Ecological Monographs, 68(1), 1–23.
- CCEF | News | Climate Change in the Coral Triangle – Dr. Alan T. White. (n.d.). Coastal Conservation and Education Foundation (CCEF). http://old.coast.ph/?q=news/climate-change-coral-triangle-dr-alan-t-white
- Harrison, H.B., Williamson, D.H., Evans, R.D., Almany, G.R., Thorrold, S.R., Russ, G.R., Feldheim, K.A., van Herwerden, L., Planes, S., Srinivasan, M., Berumen, M.L., & Jones, G.P. (2012). Larval Export from Marine Reserves and the Recruitment Benefit for Fish and Fisheries. Current Biology, 22(11), 1023–1028.
- Marsh, J. (2023). Overhunting animals: Causes, effects and potential solutions. Environment Co. https://environment.co/overhunting-animals/
Featured Ecologists:
Christine Sprunger, PhD
Dr. Sprunger studies soil health and how different factors in agriculture management can manipulate it. She studies degraded systems and how to rehabilitate them, learning how nematodes can be soil health bioindicators and how climate change impacts the rhizosphere. She has done multiple studies with Kernza, a perennial grain that she is proving can be more profitable and keep soil healthier than annual grain crops. Dr. Sprunger has found that two harvests of Kernza a year increases nutrient cycling and availability, mineralizable carbon, and provides additional revenue. She also found that established perennial crops increase the amount of carbon available to microorganisms while stabilizing the soil food web. This is very important for the climate and the environment in general. Perennial crops decrease soil erosion dramatically and help rebuild topsoil through the microorganism communities they foster. This is what Dr. Sprunger wanted to do when she came into the field. She wanted to find solutions to rehabilitate soil because she was born in Haiti and she has seen the effects of degraded soil on a third-world country. Her research is showing that agricultural management practices have the ability to foster crop fields with higher productivity without losing profitability, a major concern of many farmers.
Earyn McGee, PhD Candidate
McGee is broadly interested in research that takes place at the intersection of conservation biology and land management. She is studying the diets of three species of lizards in the Chiricahua Mountains to assess how the drying out of perennial streams can affect riparian lizard communities, which fall under the categories of both food web and ecosystem ecology. Her project involves a lot of fieldwork and “lizard lassoing,” as McGee calls it, and she uses both mark-recapture surveys and DNA sequencing of fecal matter as part of her methodologies. She is trying to determine how much the lizards rely on aquatic insects for sustenance, which could have heavy implications for wildlife inhabitants of arid environments that are being increasingly impacted by climate change. In addition to her herpetology work, McGee is also particularly interested in science communication and increasing the participation of underrepresented groups in STEM. She is a graduate student mentor for the Doris Duke Conservation Scholars Program at the University of Arizona, where she mentors undergraduate students from various backgrounds and introduces them to the intricacies of field research.
Emma J. Rosi
Dr. Emma J. Rosi studies freshwater ecosystems and how they are impacted by climate change, land use, and urbanization impact food webs. Her research has worked with contaminants such as pharmaceutical, aged wastewater infrastructure, dams, and agricultural GMOs. She also is Co-leader of a project in the Kenya reach of the Mara River researching the effects of hippos and wildebeests on food webs and ecosystems. The project is long term but research is steadily being published. One paper published in 2018 found that hippo poop can cause severe depletion of oxygen in rivers or hippo pools. Dr. Rosi’s research is important at it is about the relationship between human activity and the environment. A better understanding of our impacts can lead to a more healthy relationship with the world around us.
Student Contributors:
Note Outline: David Poling, Drake Sandman, Teddy Sarian, Raché Manning
Blog Style Summary: Sam Ellison, Steven Hart, Matthew Girouard, Roger Sandvik
Spotlight on NC: Elizabeth Mitchell, Cait Phipps, Jess Capes, Eva DeSantis
How to Apply Ecology in a Changing World: Zixuan Dong, Riley Westman, Noah Botkin
Featured Ecologists: Nicholas Terwilliger, Sasha Pereira
a species that has a disproportionate effect on the structure of the rest of the community, relative to its abundance in that community
predator populations limit the abundance of prey species, often resulting in a trophic cascade
consumers below the top level in a food web, including any organisms connected to a higher and lower trophic level
occurs when top predator populations change, causing reciprocal changes in the populations of lower trophic levels. Often results in drastic changes to ecosystem structure and functioning.
the productivity and abundance of populations at any given trophic level are limited by the productivity and abundance of populations below them (especially primary producers)
an organism that consumes both plant and animal tissues
an event that disrupts a community and clears or alters some niches (e.g., hurricane, wildfire)