Chapter 7: Predation
Vocabulary:
- Carnivore: an organism that consumes animal tissue
- Deimatic display: an otherwise defenseless organism uses a behavioral and morphological display to frighten a predator long enough to escape
- Ex: many butterflies have eyespots, and open their wings to suddenly display those eyespots when a predator is present
- Ex: blue-tongued skink flight their brightly colored tongues to trick predators into thinking they are poisonous (even though they aren’t)
- Herbivore: an organism that consumes plant or fungal tissue
- Lotka-Volterra Model: a mathematic and/or graphical representation of predator prey relationships, that assumes that the consumption of a prey species by a predator (and the predator population’s resulting size) is directly correlated with abundance of the prey species in the habitat
- Mesopredator: mid level predator species that is preyed upon by the apex predator, while simultaneously preying upon species at lower trophic levels
- Mimicry: one organism (or part of an organism) has evolved to look like another organism (or part of an organism). An organism may mimic its own species or another species.
- Automimicry: an organism evolves so that one part of its body mimics another part of its body
- Ex: some snakes have tails that look like their heads – and they may even move backward when threatened
- Batesian Mimicry: harmless species mimic deadly ones
- Mullerian Mimicry: unrelated species share a similar coloration to amplify the visible signal of a trait (e.g., toxicity)
- Automimicry: an organism evolves so that one part of its body mimics another part of its body
- Omnivore: an organism that consumes both plant and animal tissues
- Optimal Foraging Theory: foraging strategies minimize the energy spent to acquire resources, and maximize the energy gained from those resources
- Parasite: an organism that takes nutrients and energy from another living organism (called the host), but does not kill them. Parasites typically associate with their host for an extended period of time
- Parasitoid: an organism that takes nutrients and energy from another living organism, eventually killing their host
- Planktivore: true predators that specialize in consuming plankton
- Predator: an organism that consumes another organism
- Prey: an organism that is consumed by a predator
- Red Queen Hypothesis: refers to the constant “evolutionary arms race” between predators and prey
- True Predators: predators that directly hunt and kill their prey
Outline of Notes:
Types of Predation:
Predation can be divided into different categories based on what an animal eats and how it acquires its food.
- Predation can be separated into three broad categories based on diet:
-
- Carnivores: Consume animal tissues
- Herbivores: Consume plant or fungal tissues
- Omnivores: Consume both animals and plant/fungal tissues
- Predation can also be categorized by how they acquire their prey:
- True predators: Hunt and kill prey directly
- Planktivores: specialists that feed on plankton.
- Considered true predators as they consume the entire prey item, causing immediate mortality.
- Parasites: take nutrients and energy from another living organism (host) with whom they are closely associated, for an extended period of time but do not kill them.
- Not true predators as they need their “prey” to survive in order for them to remain alive.
- Parasitoids: take nutrients and energy from another living organism, eventually killing their host.
- Not true predators as they do not kill the host immediately OR consume the entire host.
adsljfsdjlf
sdfasdfsdfasdf

Question from a Student:
“I understand that animals who eat seeds cause immediate mortality (and thus qualify as true predators), because that seed would otherwise germinate and grow into a new organism. But don’t some plants rely on animals to eat their seeds to facilitate dispersal? Would this still be considered immediate mortality for the plant? How do we differentiate between these types of predation?”
Answer: Great question! It’s important to consider whether an animal is targeting the seed as their food source, or a fruiting body that houses and protects the seed, allowing the seed to pass through the animal and germinate when it’s excreted elsewhere.
- In the majority of instances where the seed is not protected and is eaten directly, the animal crunches / chews the seed, resulting in immediate mortality (and true predation).
- However, frugivores (animals who eat fruit) swallow the seed(s) whole and excrete a still-viable seed. This is an example of mutualism, since the plant and predator are both gaining something. (See Chapter 8 for more information about Mutualism.)
Modeling Predator/Prey Interactions:
- Lotka-Volterra Model: Models predator/prey interactions. Assumes that the consumption of a prey species by a predator is directly correlated to the abundance of the prey species in the habitat.
- I.e. there is a lag in the predator populations in response to the cyclic peaks of prey.
Figure 2. The Lotka-Volterra model is a cyclical model that shows the relationship between changes in predator and prey populations. In this model, the highs and lows of the predator population (foxes) mirror those of the prey population (rabbits), but with a slight lag. This model only works if there are both areas where the prey can be caught, and areas where the prey can hide. Otherwise, the predators eliminate the prey population. Created by: Marley Kelly.
- I.e. there is a lag in the predator populations in response to the cyclic peaks of prey.
- Do predator/prey cycles actually exist or is it a product of mathematical modeling?
- Natural heterogeneity in the environment allows for recovery of prey as well as predator populations, which shows the cyclical nature of these dynamics.
- The greater number of prey, the more the predators can eat.
- Three types of consumption curves:
- Type 1: Linear; predators consume the same proportion of prey, regardless of prey abundance
- Ex: Filter feeders
- Type 2: Linear in a decreasing fashion then reaches a plateau; predation declines as prey abundance increases
- Ex: Lynx are efficient hunters that quickly spot prey but are limited by handling time.
- Most common type of consumption curve
- Type 3: Sigmoidal curve with low consumption when prey are too sparse, highest rate of predation at intermediate proportions of prey abundance, and plateaus at an asymptote similar to Type 2.
- Ex: Crabs may switch prey items if one is too scarce; and are also limited by handling time.
- Type 1: Linear; predators consume the same proportion of prey, regardless of prey abundance

Predator Responses:
- Predators respond to prey availability functionally and numerically:
- Functional response: relationship between the rate of consumption and the number of prey available
- Increased prey abundance = increased predator consumption
- Numerical response: relationship between the rate of consumption and the number of predators in an area
- Increased consumption = increased predator populations
- Predator populations can increase via reproduction and immigration
- Game Theory/Optimal Foraging Theory: time and energy are considered in foraging
- Natural selection favors efficient foragers:
- Maximum energy or nutrient intake per unit of effort
- Minimize time/energy spent foraging
- Factors to consider:
- Type of prey item
- How to search for prey
- Location and amount of time spent searching
- Ex: Pied wagtails preferred medium-sized prey because it maximized calories and minimized time spent eating (Davies 1977)
- Natural selection favors efficient foragers:
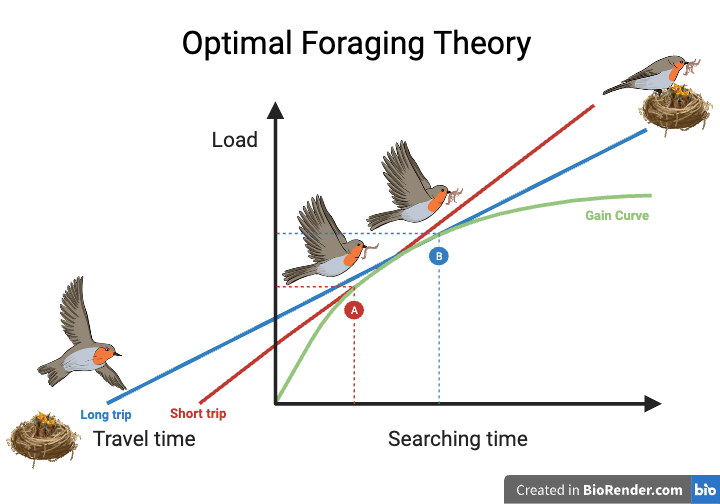
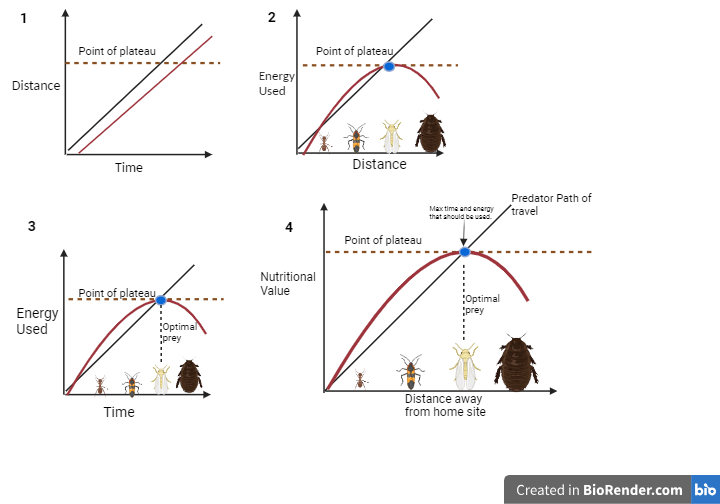
Avoiding Predation:
- Coevolution involves 2 “players”, both constantly changing in response to the other’s changes. The “players” could be two species, male(s) and female(s), 1 species and the broader ecosystem, etc.
- Red Queen Hypothesis: constant evolution and adaptation is needed for a species to maintain its fitness relative to the other species or systems it is interacting with
- Examples: colors, camouflage, venom, poison immunity, etc
- How do prey species avoid predation?
- Two main defense categories:
-
-
- Induced: brought on by predators
-
-
-
-
- Ex: Behavioral defenses (e.g., alarm calls, intimidation, playing dead)
-
-
-
-
- Constitutive: permanent fixed features
-
-
-
-
- Ex: Camouflage, mimicry, protective armor, satiation of predators (e.g., mass emergence of cicadas)
- Mullerian Mimicry: Unrelated species share a similar coloration → amplifies signal
- Batesian Mimicry: Harmless species mimic deadly ones
- Ex: Camouflage, mimicry, protective armor, satiation of predators (e.g., mass emergence of cicadas)
-
-
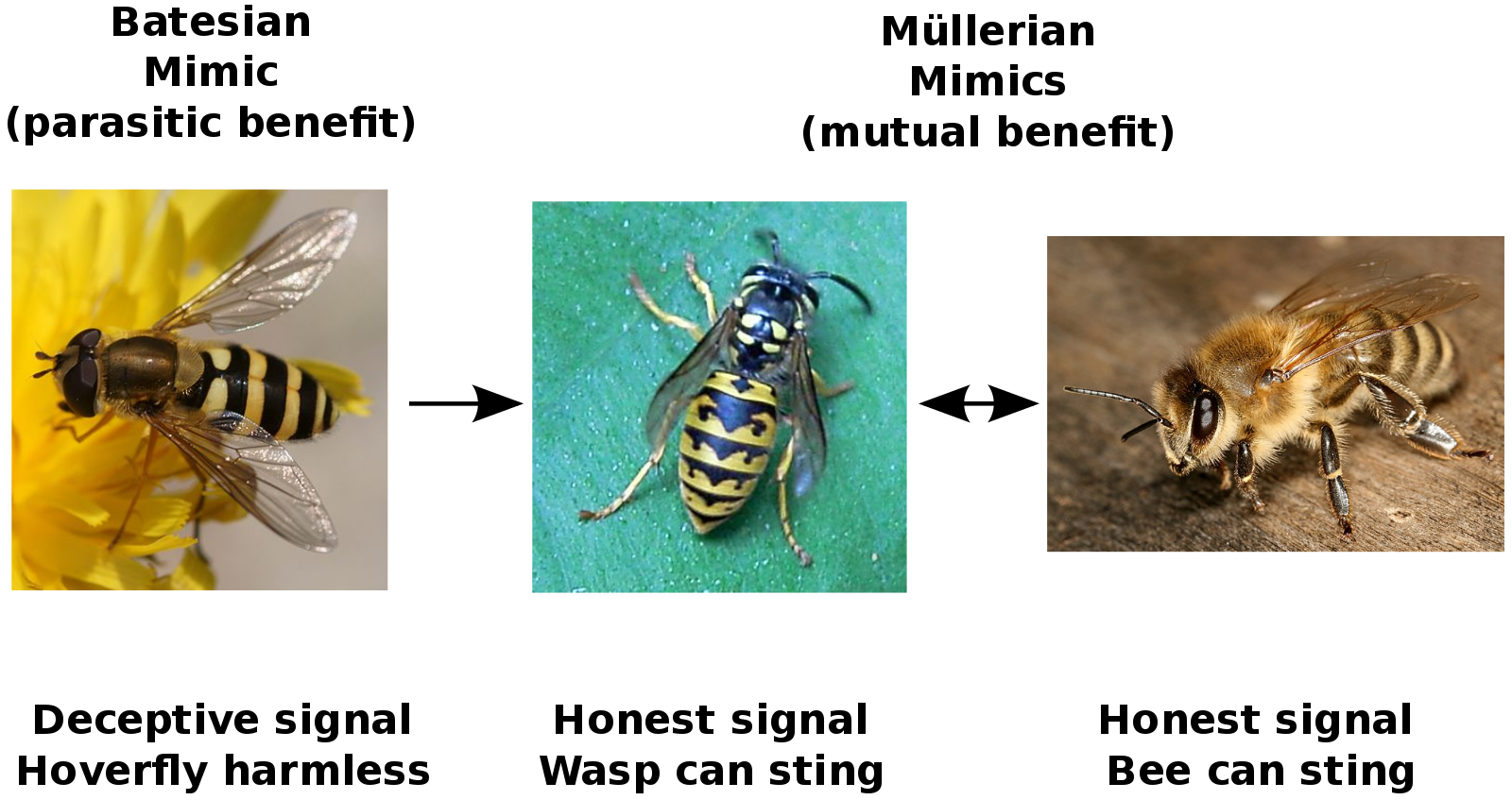
- How do plants avoid predation? (Note: a plant may use many modes of defense simultaneously.)
- Structural Defenses:
- Ex: thorns, seed shells, trichomes
- Chemical Defenses:
- Active inhibitors: chemical compounds released in response to damage, functioning as a cry for help (induced)
- Quantitative inhibitors: a compound produced in large quantities
- Ex: tannins, resins, fiber (which also provides structural defense because it is difficult to chew and impossible for animals to digest)
- Phytoestrogens: chemicals that disrupt reproduction
- Qualitative inhibitors: small amount will substantially affect predator
- Ex: nicotine, caffeine, spicy compounds (e.g., capsaicin)
- Relationships with animals that protect the plant
- Ex: bullet ants protect host tree
- Structural Defenses:

Interactions Between Plants, Herbivores, and Carnivores:
Plant, herbivore, and carnivore densities all interact and affect one another.
- Predators influence prey dynamics by inducing morphological, behavioral, and physiological defense responses.
- Herbivore costs of avoiding predation:
- Loss of feeding opportunities
- Reduced foraging time and food intake
- Delayed growth/development
- Decreased population size
- Potential costs of predation
- Injury
- Dehydration
- Negative interactions with other predators
- Time spent away from offspring
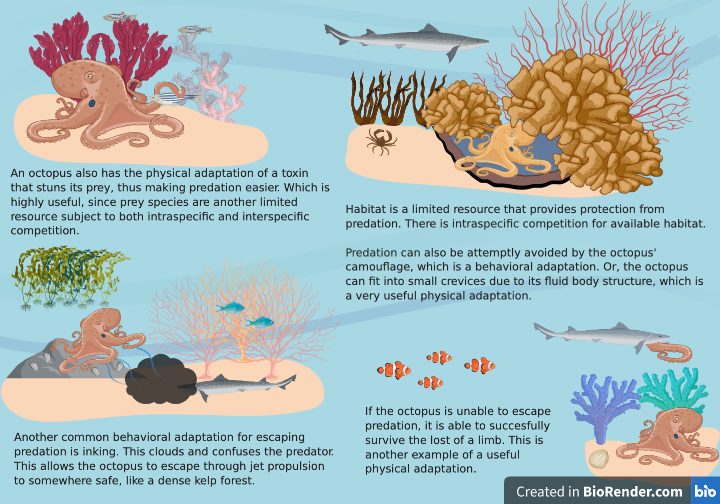
Key Takeaways
Overarching Themes and Unifying Concepts:
- Predators don’t have to just consume meat. They can eat:
- Plants (including seeds)
- Animals (including eggs)
- Fungi
- Any living organism
→ But only true predators kill their prey upon consumption (e.g. a grazer is not a predator, but an animal that eats the entire plant is).
- Predator-prey relationships can be used to map populations and their health
- If the deer population plummets, expect the population of wolves to crash soon after.
- Keeping track of one species’ population can tell you a great deal about its predators’ population.
- Depending on their predator’s pattern of consumption, prey utilize different modes of protection/defenses.
- Defenses are not just limited to animals, plants have defenses as well.
Blog-Style Summary:
When you think of predation, you probably imagine lots of different things, maybe it’s wolves eating rabbits or sharks eating fish, but there are many ways that predation occurs in the ecological world. Starting with the basics, a predator is an organism that feeds on and consumes other organisms. But what types of predators are there? Predators can be defined by the type of prey that they consume. Carnivores are organisms that consume animal tissue, such as tigers (seen in figure 4). Herbivores consume plant or fungal tissue, like rabbits (seen in figure 5). It may seem shocking that herbivores are considered predators, but believe it or not they are since plants are still considered prey items! Lastly, we’ve got omnivores, which are organisms that consume both plant and animal tissue, and a classic example is the grizzly bear (Ursus arctos horribilis) (seen in figure 6).
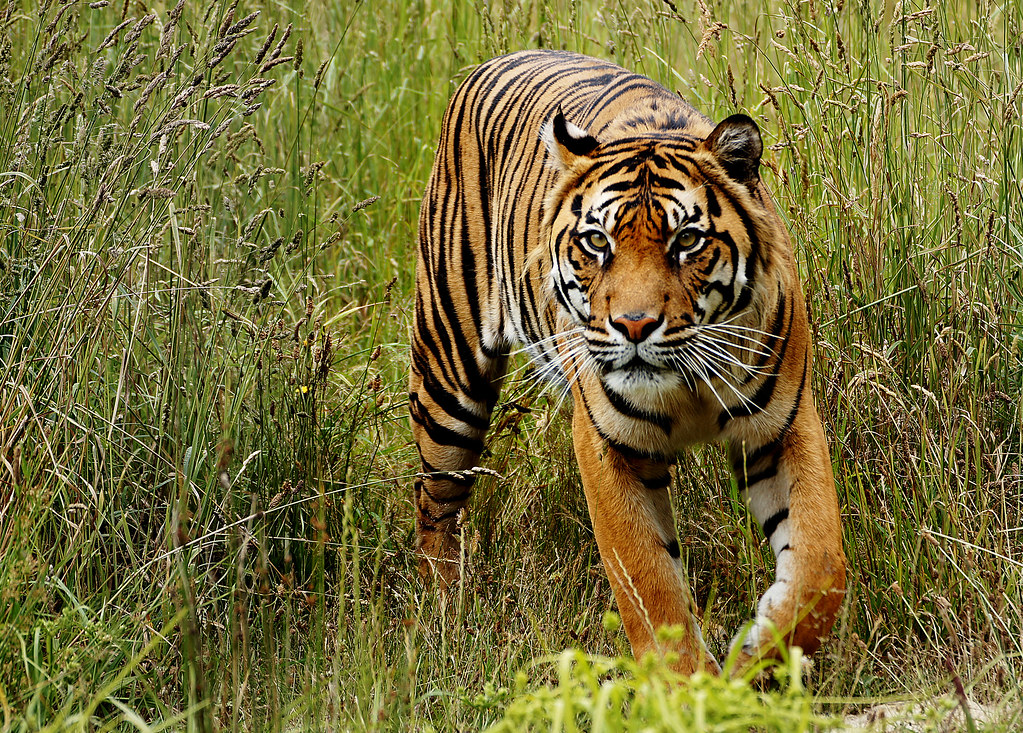
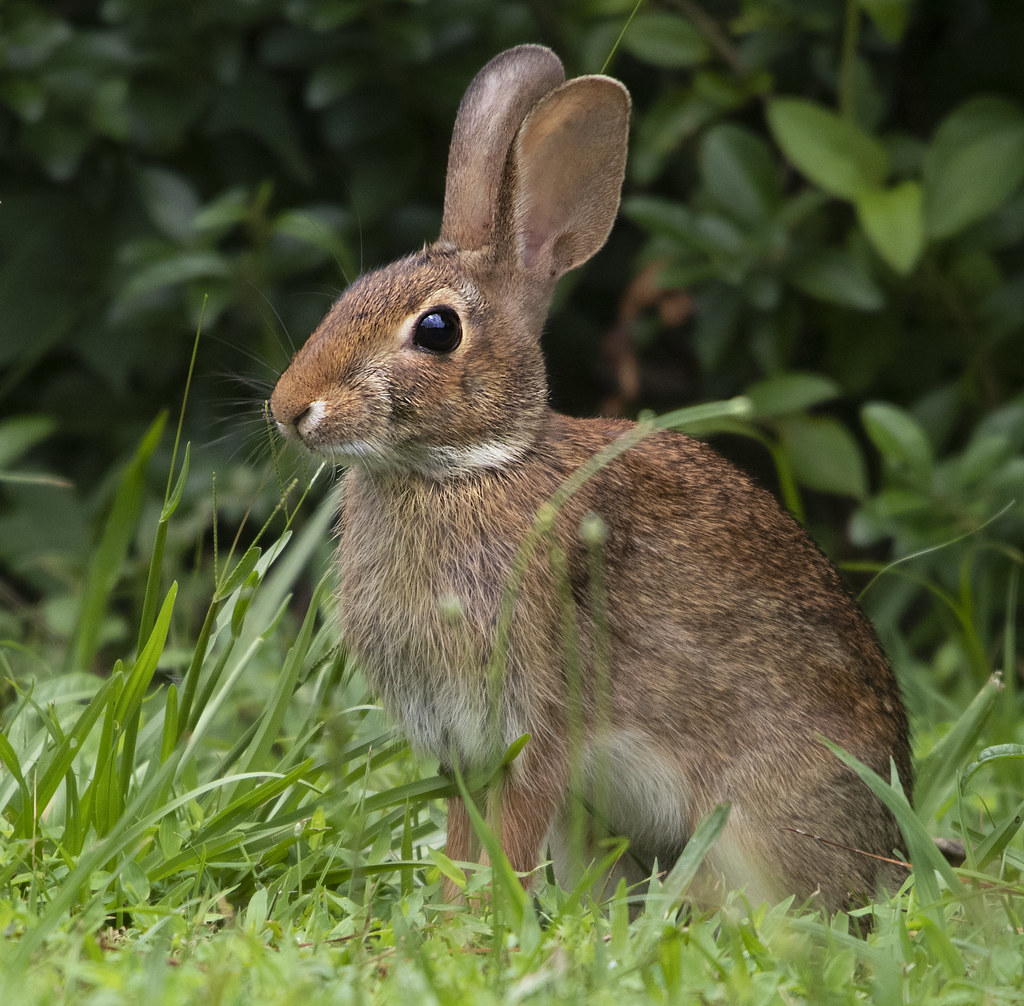
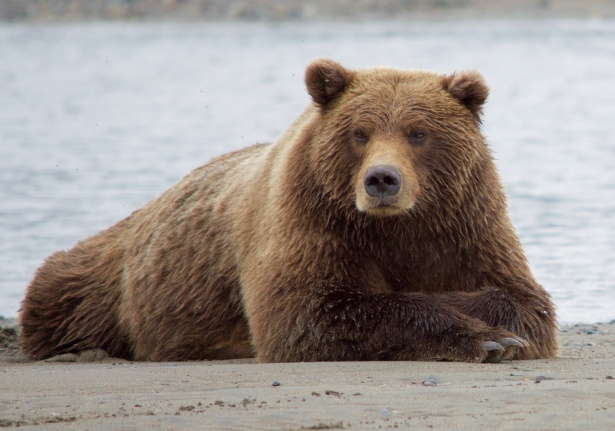
But what makes a predator a “true predator”? Usually, the traits that determine what a true predator is are whether or not they kill their prey immediately and consume them entirely. So species that are grazers, browsers, or scavengers (feeding on part of each victim without actually killing it outright), parasites (feed on their host while it’s still alive and are not immediately lethal), or parasitoids (take a long time to kill their prey) don’t fit this definition and are therefore not considered true predators! Just to solidify this concept, let’s go over some examples of what would be considered a true predator. Seedivores, or animals that consume seeds, are considered true predators; their ‘prey’ is entirely consumed and (most of the time) does not survive being eaten. An example of this is a black-capped chickadee (Poecile atricapillus) eating a sunflower seed. Another category of true predators includes planktivores, which are species that consume zooplankton and/or phytoplankton. An example of a planktivore is a whale shark (Rhincodon typus); this species eats its prey by opening its large mouth and slowly swimming through swarms of plankton on the ocean’s surface (Seen in Figure 7).
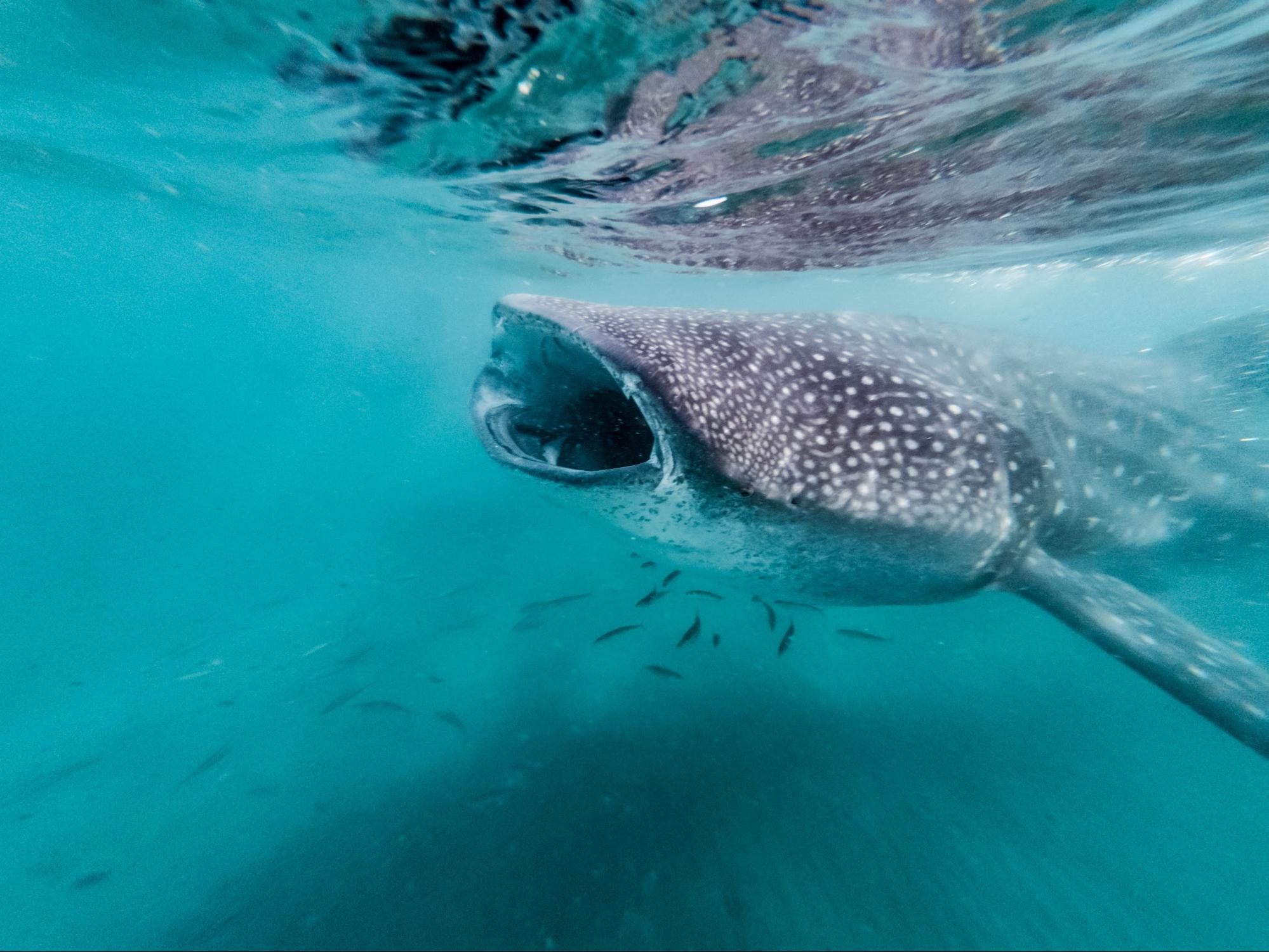
Predators can respond to prey availability in two ways: functionally, through their rate of consumption, and numerically, through their change in population densities. If prey densities are high, a predator’s rate of consumption will increase (functional response) and their reproductive rates will increase, increasing predator populations (numerical response). In order to understand these complex predator-prey interactions and their effects on the species involved, we often look to the Lotka-Volterra Model
(See figure 8). We use this model to consider the population dynamics of two different species at the same time. Following the trends of the chart, predator populations lag behind the population peaks of prey. It can be important to consider this model when predicting the effects of one species on another. For example, if lions completely disappeared from the Serenegeti, how may this affect Thomson’s gazelle populations? The answers to these questions are more easily predicted using the Lotka-Volterra model, which can give indications of species population health. Many factors influence control of prey populations by predators; this includes availability of cover for prey to hide, choice of multiple prey species, prey scarcity and evolutionary changes in predator and prey species.
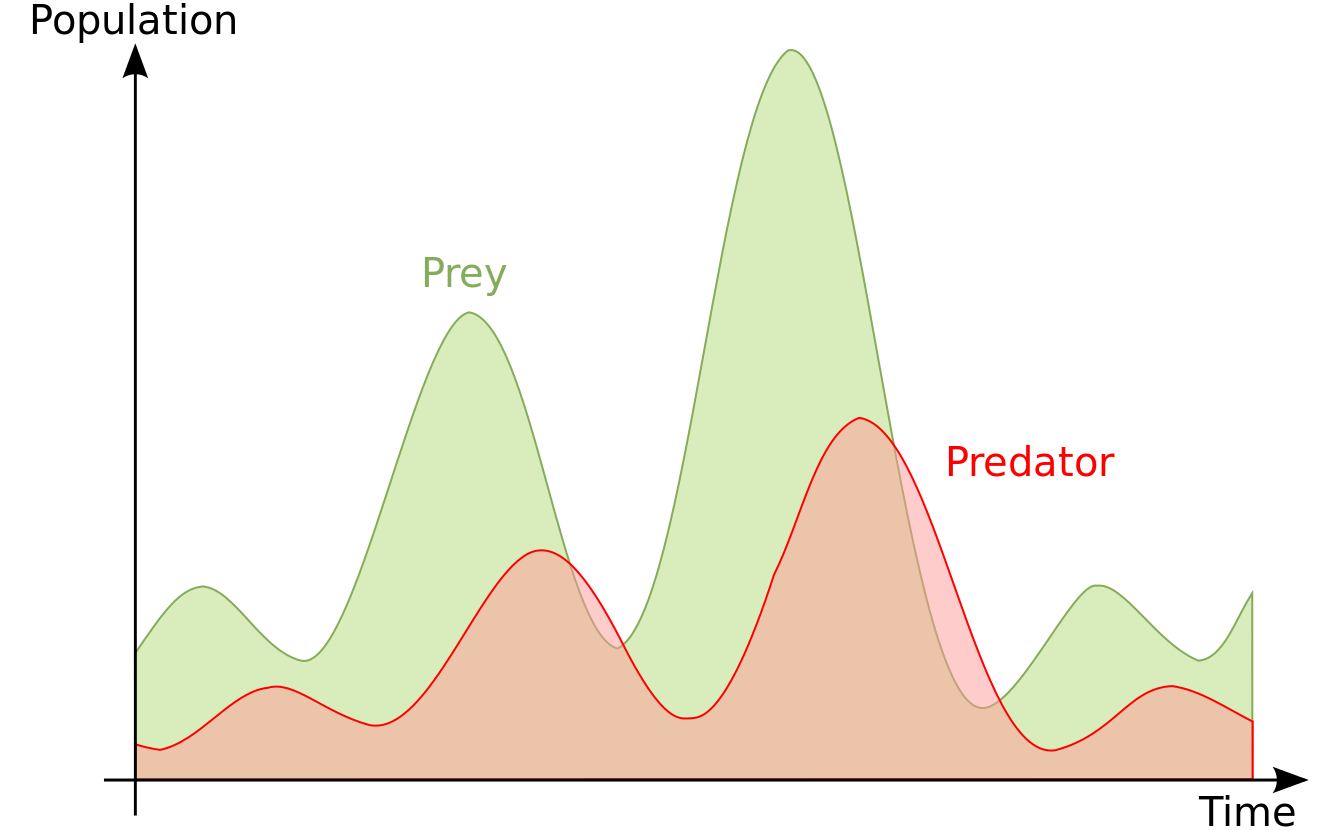
This model is a good basic example of how predator and prey populations play off each other, but some scientists in the past have questioned how accurate it is in the years after it was created. In one study, headed by C.F. Gauss, the “predator lags behind prey” dynamic was only seen when predators and prey were added to already existing populations in his tests. But this study was really simple, and one by C.B. Huffaker took these tests to the next level. Huffager studied orange mites, and after setting up his experiment… they were all killed by predation. However, when partitions and shade were added to allow prey to hide, the Lotka-Volterra model predictions were observed (Figure 9)! Having areas for prey to hide (but also areas where they can be caught) was the key. The Lotka-Volterra model isn’t just purely mathematical – it’s been proven to exist in the field.
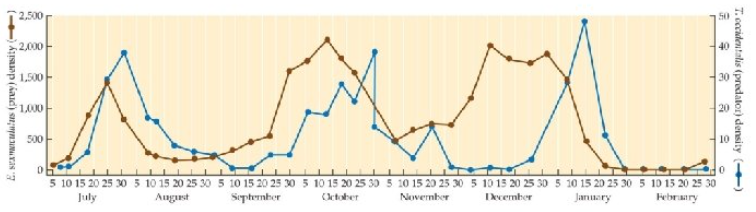
So when predators aren’t eating their prey, what are they doing? At some point, they need to increase their population size! Predators do this through reproduction and aggregation, which is the collection of predators coming together through immigration. When predators are searching for prey, they must sacrifice time and energy. According to the Optimal Foraging Theory, or Game Theory, predators must utilize their limited time and energy as efficiently as possible to survive. Therefore, natural selection favors efficient foragers; predators must maximize the energy/nutrient intake per unit of effort, and minimize the time and energy spent on foraging. An example of an efficient forager includes the pied wagtail (Motacilla alba), which prefers medium-sized prey species to minimize handling time and maximize caloric intake (Figure 10).
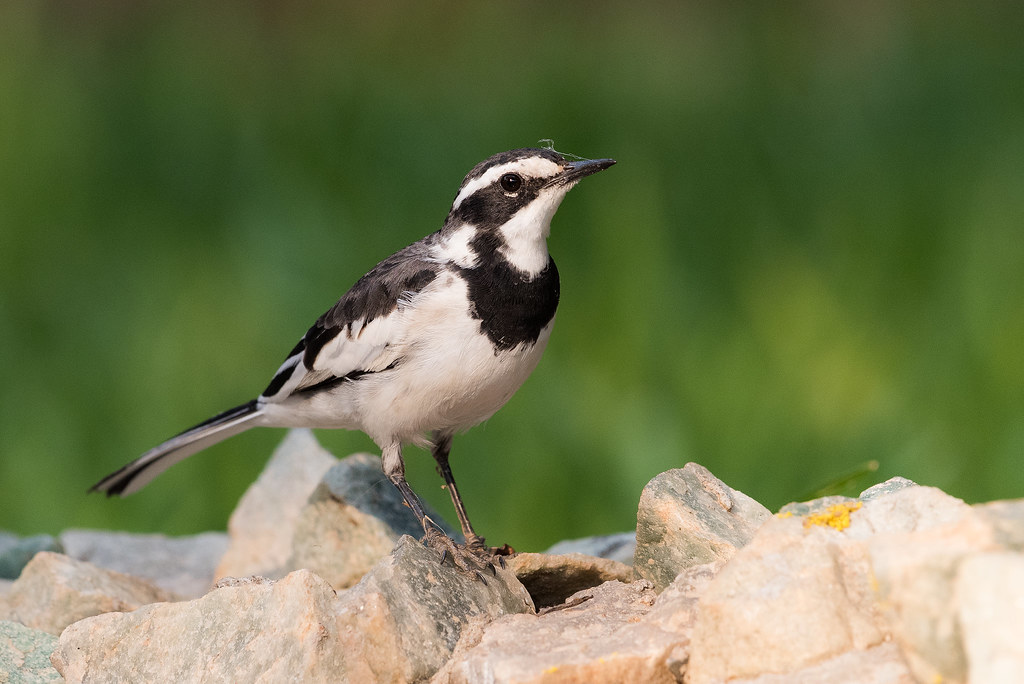
Considering how complicated predation is, it’s no surprise that it has effects on conservation. When conserving true predators or prey species, the dynamics of predation are always important to consider. Giving predators access to prey usually isn’t enough. In some species, like the African Wild Dog (Lycaon pictus) (Figure 11), prey needs to be available for them to eat, but not so much that lions and hyenas begin to frequent the area, since they will chase the dogs away from their prey in order to scavenge it for themselves (Creel, 2001).
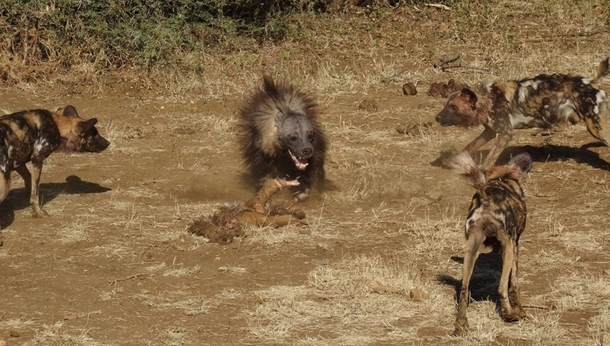
Looking at predation and our ‘true predators’, we can see just how important predation is, especially in environments where we find intraguild predation, when natural competing predators prey upon one another while simultaneously preying upon other species in the area. This type of scenario leads to areas that are bound to have mesopredators as well. Mesopredators are the mid level predators that are preyed upon by the top level predator, while simultaneously preying upon the lower level species. Mesopredator issues arise in environments where the top level predators are removed by natural or human causes. The absence of top level predators will lead to a major increase in the mid level predators, to the point of an overabundance. (Muller & Brodeur, 2002). Through a classic snowball effect, we will be left with a diminishing population of prey species, and an overpopulation of these mesopredators. Along with this, we can look at areas where there is a lack of predator species altogether. Certain residential areas that contain an overpopulation of white-tailed deer see a major increase in vehicle collisions. When you add in predators, deer populations will even back out and deer collisions become much less of an issue. Overall, we can see that predator-prey relationships can be a very complicated thing to look at. There are many factors that contribute to the abundance of both, as well as many factors that contribute to the overall health of an ecosystem. Predation is a key factor in ecosystem health, and is something that is absolutely necessary to promote positive conservation practices.
Spotlight on NC:
Did you know that on your drive to the popular vacation spot, the Outer Banks, you can hear the wild howls of “America’s wolf”? Red wolves (Canis rufus) are an historically important predator, which means they consume other organisms, known as prey. More specifically, they are carnivores, meaning they consume animal tissue. They commonly hunt rabbits, raccoons, mice, nutria, and white-tailed deer [1]. Their history in America and more specifically, North Carolina is complicated and their role as wild predators is an important conservation issue.
Early in America’s history, red wolves were hunted and killed by European settlers due to fearful and competitive attitudes that drove predator eradication programs. By the 1960s, only a few were left in the wild in Texas and Louisiana, and 17 pure red wolves were identified to begin a captive-breeding program. This unique species was considered extinct in the wild in 1980 with barely over 60 in captivity, but a wild population was established in 1987 by the U.S. Fish and Wildlife Service (USFWS) [2]. The USFWS chose the North Carolina coast, specifically Alligator River and Pocosin Lakes National Wildlife Refuges, to establish this managed population because it already had large amounts of protected land, was less developed, and coyotes had not expanded into that area at the time. This landscape is a productive natural system with a notable amount of biodiversity and differing landscape types such as agricultural, developed, undeveloped, and state/federal land [2]. Additionally, this region is an ecologically unique area with swamps, forests, coastal prairies and rare pocosins which provides varying habitat and resources for the wolves [1].
The population of red wolves established in the National Wildlife Refuges is considered a nonessential-experimental population (NEP) under Section 10 of the Endangered Species Act, which is a legal term that means that the individuals that are released are protected and if they are not successful we would not lose valuable genetics. In the early 2000s, the number of estimated wild red wolves was believed to be 130, which is when the North Carolina population peaked. Since then, there has been a notable, slow decline in the population size [2]. Human interactions, like gunshot mortality and vehicle strikes, coyote hybridization, and program changes by the USFWS have affected the trajectory of population recovery of the red wolf [1, 2]. Currently, wild red wolf populations are estimated to be around 20 individuals.
Red wolves act as a control agent for the prey populations in their territory. They especially regulate deer and “nuisance” populations, such as raccoons and nutria, which prevents them from destroying cropland and other human resources [1]. They are true predators that hunt and kill their prey immediately upon capture. White-tailed deer consist of over half their diet and are important prey species to the wolves. A further breakdown of the composition of the red wolf diet can be seen in Table 1, and highlights their unique ecological role in NC food webs [3].
Wolf consumption of deer is extremely important for population control. Because deer are herbivores, meaning they consume plant or algal tissue, the overpopulation of white-tailed deer in the absence of wolves in North Carolina has led to ecological problems such as the overgrazing of vegetation. Overpopulation has also led to increased negative human-wildlife interactions such as vehicle strikes, and negative impacts on other small mammal species. The removal of native predators, namely the red wolf, that regulate these populations has caused populations to skyrocket. With the rise of diseases in prey species, such as Chronic Wasting Disease, the re-establishment of natural predators is extremely important to recover and improve native ecosystems in North Carolina [4].
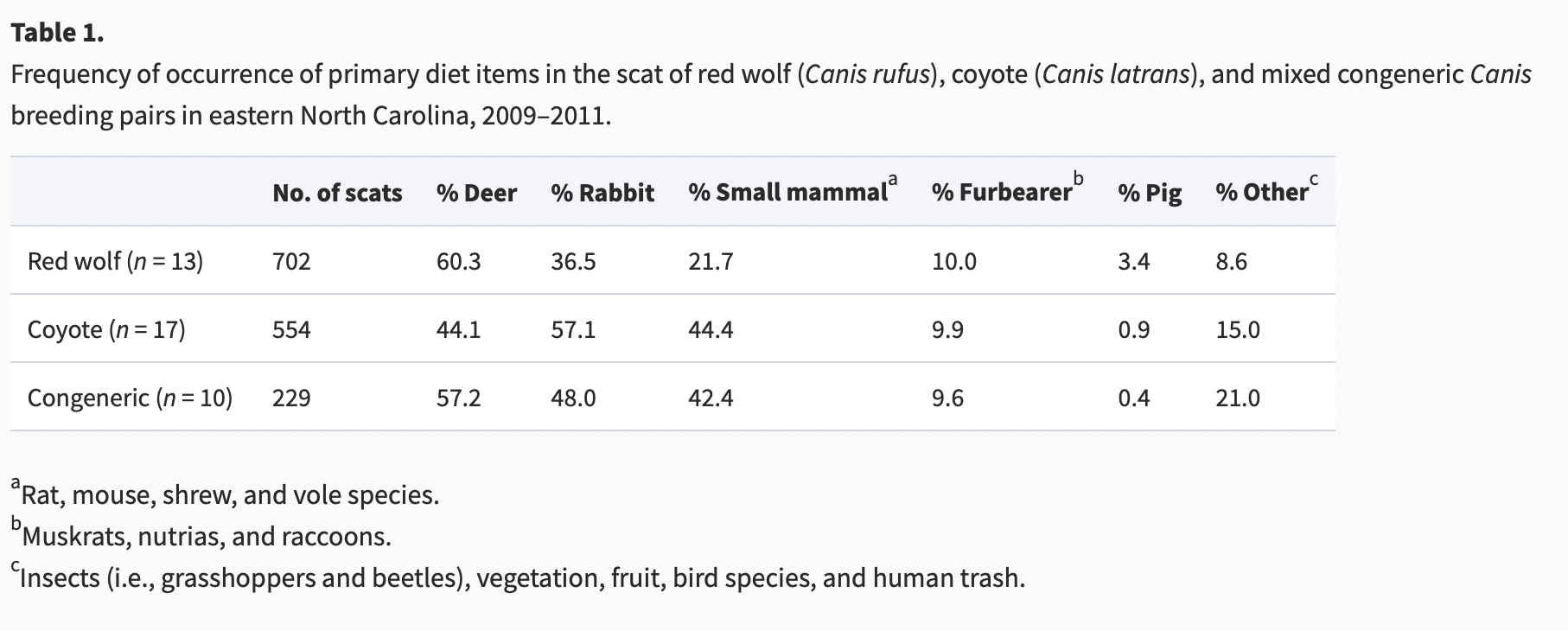
Red wolves also consume other herbivores and prevent their populations from growing too large and consuming all of the nutrients available in the ecosystem. Red wolves influence prey dynamics in their region by influencing prey defense responses, and a lack of these natural predators can fundamentally change the behaviors and landscape interactions of these prey species. Predator-prey dynamics, when there are enough of each species for this to be significant, allows each to regulate the other’s population with both numerical (increasing rate of prey consumption leads to increased predator population) and functional responses (the rate of consumption based on the number of prey). However, due to their current population sizes being so low and very high mortality rates, the red wolves are not able to increase their population size to fully follow a predator-prey model. There are no other populations for individuals to aggregate, or immigrate, from and although there have been some pups born there is an unfortunate lack of breeding pairs.
The conservation and management of red wolves provides a unique reminder of how human disturbance and habitat fragmentation impacts the fields of ecology, biology, and conservation. As biodiversity levels continue to decline and as urbanization and habitat fragmentation continues to increase around the globe, protecting and understanding predators, like the red wolf, will become increasingly important. Predators heavily influence their surrounding natural systems (even your nearby environment!) and their ecological roles are undoubtedly important. Restoring this NC predator could help re-introduce a healthy predator-prey dynamic as well. Public education on the importance of predators to natural systems and the beneficial role they often play for humans is vital to continue conservation efforts.
How to Apply Ecology in a Changing World:
Everybody has to eat, right? Most people are aware of the roles of predators and prey. Predators are the ones that eat what they catch and the prey are the ones being eaten. A picture of a fox chasing a rabbit may come to mind or even a lion chasing a gazelle but, what about a whale eating plankton? Or coral that filters their food from the surrounding water? Or even a goat eating grass? These animals are technically predators even though their feeding strategies may seem more passive and non-threatening to us. A predator is simply any organism that consumes another organism. Some predators prefer plants only, and those are our herbivores. Others prefer meat only, and these are the carnivores. Finally, we can’t forget about the middle ground, which are omnivores. They come into play by eating a mix of plant and animal tissues. These may seem very different, but rest assured they are all different types of predation! A “subclass” of predators, true predators, refers to any organism that consumes its entire prey at once, causing immediate mortality. True predators are the ones that people think of most often as predators.
How do predators find their prey in open areas? This is a grand challenge associated with predators who are constantly in pursuit of their next meal that they directly hunt and kill. Picturing an open landscape like a desert or an open ocean may make you wonder how anyone could locate their next meal, but predators have adapted in order to survive in these conditions. For example, toothed whales use biosonar to locate their prey in complex environments [1]. This means that they hunt by sending signals and listening for their echoes (when they bounce off of prey). Another large ocean predator is the manta ray. They are planktivores that swim through the water and filter out all the plankton to eat. They certainly don’t have to do as much active searching as the toothed whales. Another unique method is practiced by anglerfish, who dangle a bioluminescent light in front of their face as a lure to attract unsuspecting prey [2]. Just as predators have adapted to hunting prey, prey evolve defense mechanisms to avoid being eaten. The Red Queen Hypothesis states that the relationship between predators and prey is an “evolutionary arm’s race” (Figure 17) [6]. Prey have developed many defense mechanisms, including Mullerian and Batesian mimicry. Mullerian mimicry is when two or more organisms evolve a similar appearance to signal their toxicity to predators, whereas Batesian mimicry is when one palatable species mimics the appearance of a non-palatable species to lessen its chances of predation [6].

Humans interfere with predators’ ability to find and catch prey in many different ways. One of the main ways humans interfere with the predator-prey relationship is by modifying landscapes [4]. When humans modify landscapes this can decrease vegetation, which will also decrease the population of prey animals that rely on vegetation for food or shelter. As prey populations decline, the predator population would decline as well. By contrast, logging can be very beneficial for predators. Logging creates cutblocks and roads [4], increasing predators’ travel efficiency and decreasing prey cover, making it easier to find prey in smaller habitat patches. The Optimal Foraging Theory comes into play here: if there is not an optimal amount of food for an animal, they will migrate to a location with more resources [5]. This means that, while predation efficiency is higher in logged areas, many prey species may ultimately emigrate to larger patches with more resources; and predator populations will follow.
Human interference with predation and prey relationships usually favors the prey species. Predators need more space and resources relative to their body size to survive, whereas small prey populations are better able to integrate with increasing human populations. Urbanization shrinks the area that large predators are able to inhabit and decreases their ability to survive [6]. Think of squirrels and bears, for example: the squirrels are able to find food and shelter within human habitats, without humans retaliating to the “nuisance”. (In comparison, bears who “loot” dumpsters or other anthropogenic food sources, especially on private property, are often relocated or killed.) At the same time, many large predators avoid human disturbance, making high-traffic areas a refuge for smaller prey like squirrels. Some solutions to reduce human interference in predator-prey relationships would be to decrease urban expansion and limiting impervious gray spaces such as roads and highways that intersect major natural open spaces [6]. Humans’ exponentially growing population is affecting all kinds of different landscapes and ecosystems, with different consequences at different trophic levels.
References
- Jensen, F. H., Rocco, A., Mansur, R. M., Smith, B. D., Janik, V. M., & Madsen, P. T. (2013). Clicking in Shallow Rivers: Short-Range Echolocation of Irrawaddy and Ganges River Dolphins in a Shallow, Acoustically Complex Habitat. PloS One, 8(4). https://doi.org/0.1371/journal.pone.0059284
- Monterey Bay Aquarium (2023) Deep-sea anglerfish. Monterey Bay Aquarium. https://www.montereybayaquarium.org/animals/animals-a-to-z/deep-sea-anglerfish#:~:text=Anglerfish%20have%20perfected%20the%20art,the%20anglerfish%20snaps%20it%20up.
- Ward, S. A., Leather, S. R., Pickup, J., & Harrington, R. (2001). Mortality during dispersal and the cost of host-specificity in parasites: how many aphids find hosts? Journal of Animal Ecology, 67(5). https://doi.org/https://doi-org.prox.lib.ncsu.edu/10.1046/j.1365-2656.1998.00238.x
- Boucher, N. P., Anderson, M., Ladle, A., Procter, C., Marshall, S., Kuzyk, G., Starzomski, B. M., & Fisher, J. T. (2022). Cumulative effects of widespread landscape change alter predator–prey dynamics. Scientific Reports, 12(1), Article 1. https://doi.org/10.1038/s41598-022-15001-3
- McKenney, E. (2023). Chapter 7: Predation. Pressbooks: Applied Ecology https://ncstate.pressbooks.pub/appliedecology/chapter/chapter-7-content-predation/
- Muhly, T. B., Semeniuk, C., Massolo, A., Hickman, L., & Musiani, M. (2011). Human activity helps prey win the predator-prey space race. PLoS ONE, 6(3), e17050. https://doi.org/10.1371/journal.pone.0017050
Featured Ecologists:
Lauren Esposito
Lauren Esposito is an arachnologist at the California Academy of Sciences who specializes in scorpions. Her research focuses on the evolution and biogeography of scorpions in the Buthidae family, and a lot of her work focuses on species found on several Caribbean islands. She also studies the properties and genetics of scorpion venom, and some of her research in this area has been used in the context of cancer treatment research. Additionally, her research on venom genetics in striped bark scorpions relates to our unit on predation, because they are in an evolutionary arms race with one of their predators, the grasshopper mouse. Since grasshopper mice have evolved to withstand the sting of a scorpion, these scorpions will need to evolve further overtime to have more potent venom that will paralyze or kill the mouse. Esposito is studying the genes that affect venom toxicity in scorpions, of which there are an estimated 200+ unique genes. In 2017, she aided in the discovery and naming of three species of clubbed scorpions, and in 2018 she discovered a new species of ghost scorpion. Dr. Esposito is also the co-founder of two nonprofit organizations dedicated to science education and the amplification of underrepresented voices in science, Islands and Seas and 500 Queer Scientists.
Corina Newsome
Corina Newsome studied the impacts of predation and climate change on where seaside sparrows choose to locate their nests, how distributed their populations are, and how successful their populations are. Climate change has caused increased sea level rises, leading to more often flooding within marshes–the seaside sparrows primary nesting environment. This increased flooding causes the sparrows to nest on higher elevations, as shown in the middle of the Biorender. However, nesting at this height increases the risk of predation due to less vegetative cover. Sometimes these marsh habitats are located near roads, causing nesting sites to be on roadsides. These areas experience less flooding due to a further distance from the marsh wetlands or rivers, however, they experience increased predation due to ample access for predators like raccoons. In Corina’s research, they discovered that despite nesting on higher land or near roadsides increasing predation, seaside sparrows often pick this risk over the risk of nest flooding from lower elevations. Therefore, edge habitats created from sea level rising due to climate change have more of an impact on seaside sparrow nesting sites and fitness than predation. This study relates heavily to our topics of predation and habitat fragmentation, and how those relationships impact the overall fitness of a species. Although this study did not agree with the original hypothesis, it is still useful information that can be used in future predation management considerations.
Corina Newsome’s graduate research focuses on illustrating the impact of climate change and predation on MacGillivray’s seaside sparrows. These sparrows nest in the marsh grasses, thus they are extremely susceptible to flooding from rising sea levels. This frequent flooding forces the birds to build their nests higher in elevation on the coast, making them more vulnerable to predators like mink, rice rats, and raccoons. This project incorporates issues on the population level (predation and population densities), but it also applies global issues as well. Climate change impacts many different populations, not just the seabirds. Newsome’s project is focused on understanding the relationships between these threats and the fluctuating population sizes of seaside sparrows. This will hopefully lead to more information that can help to optimize wildlife management. Implementing management strategies such as building structures to shield more vulnerable nesting sites will help to support the seabirds reproductive efforts. Outside of her research, Newsome is a strong advocate for diversity within wildlife and conservation. She helped to co-organize “Black Birders Week” last year, which highlights an underrepresented group of researchers in the field.
Terrie M. Williams, Phd
Dr. Williams’ research focuses on the physiology of large predators and how it relates to human physiology. Her lab, Williams ICE (Integrative Carnivore EcoPhysiology) Lab at University of California, Santa Cruz studies the relationship between humans, animals, and their environment to understand physiological adaptations. Her work relates to what we have studied in class about adaptations and evolution. One of Dr. Williams’ studies took place in Antarctica, where her team is researching Weddell seals and how they may follow magnetic fields underwater. The goal of this study was to understand how the Weddell seals are able to return to the same ice hole after hunting underwater hundreds of meters below the surface and in the darkness of winter. They hypothesized that the seals have a sort of “sixth sense” that allows them to follow magnetic fields. Her team studied this by using specially designed cameras that they attached to the top of the seals’ heads which recorded their underwater activity and tracked multiple factors. They found that the swimming behavior of the seals resembled the pattern of homing pigeons that flew around magnetic lines. This is really interesting because it is the first evidence of a marine mammal following magnetic lines. Dr. Williams also has a book titled “The Odyssey of KP2” about her fight to save the endangered Hawaiian monk seal.
Nyeema Harris, PhD
Dr. Harris broadly studies carnivore movement, distribution, and interactions with humans. She is interested in how the presence of apex predators affects the behavior and distribution of mesopredators, and how human presence can induce similar fear-based behavioral responses in wildlife species.
Alex Jensen
In Alex’s coyote diet research, Alex asked the question “How does geographic and ecological patterns during range expansion affect coyote diet”. To do this he and his team used previous research on coyote diet in different regions and also at different seasons, and concluded that coyotes were more carnivorous in temperate forests than in other regions. This is because of the greater ungulate consumption. Coyote diet was more diverse in the spring, which is where human footprint was greater. The study also found out that larger coyotes ate larger prey. Also coyotes preferred hunting instead of scavenging. With this research we can see how coyotes affect trophic levels and other ecosystem functions. He was looking at predator-prey relationships, which is what we learned in class. Other projects working he is working on, is researching what diverse students would need for them to be able to do research. Most students said they would need a higher pay and an inclusive environment.
Yannis Papastamatiou, Phd
Dr. Papastamatiou focuses on shark behavior ecology by using tagging technology on a range of sharks such as oceanic whitetips and reef sharks. He also studies predator ecology which entails focusing on what drives species habitat selection and movement, studying the physiology of digestion in sharks, and how fish transfer nutrients across habitats. To study predator ecology he uses a variety of methods such as chemical tracers, multi-sensor data loggers, telemetry, videos, stable isotope analysis, stomach content analysis, and biologgers. Dr. Papastamatiou looks for the answers to what drives predator-prey relationships and looks to conserve sharks through their research and aiding in the reduction of shark fin trade. Not only does he focus on predator-prey relationships but also overall shark behavior and distribution, for example, he is studying the ability of elasmobranchs to reproduce asexually in the wild through DNA analysis. This research would be used for shark conservation to help improve management strategies of threatened shark and ray species and ecosystems. Recently he has discovered that some shark species have long-term social bonds. Dr. Papastamatiou has been on National Geographic, BBC, and Discovery’s Shark Week to display his research on shark ecology.
Student Contributors:
Note Outline: Annika Preheim, Davis Grubb, Jared Lamb, Tommy Fairbairn, Katherine Abbott
Blog Style Summary: Lauren Willhite, Molly Niekamp, Evan Storie, Ally Peters
Spotlight on NC: Rhianna Klewin, Ren Rooney, Rachel Weaver
How to Apply Ecology in a Changing World: Ashley Hunsucker, Katie Savant, Mia McDowell, Kylan Rivera
Featured Ecologists: Sydney Beck, Mayla Ngo, Lucie Ciccone, Alana Brown
an organism that consumes another organism
an organism that consumes animal tissue
an organism that consumes plant or fungal tissue
an organism that consumes both plant and animal tissues
a predator that directly hunts and kills their prey
an organism that takes nutrients and energy from another living organism (called the host), but does not kill them. Parasites typically associate with their host for an extended period of time.
an organism that takes nutrients and energy from another living organism, eventually killing their host
true predators that specialize in consuming plankton
a mathematic and/or graphical representation of predator prey relationships, that assumes that the consumption of a prey species by a predator (and the predator population's resulting size) is directly correlated with abundance of the prey species in the habitat
foraging strategies minimize the energy spent to acquire resources, and maximize the energy gained from those resources
mid level predator species that is preyed upon by the apex predator, while simultaneously preying upon species at lower trophic levels
an organism that is consumed by a predator