Chapter 2: Life History Strategies
Vocabulary:
- Altricial: offspring are born less developed, requiring significant amounts of parental care
- Asexual reproduction: produces genetically identical offspring
- Dioecious: plants that have either male or female reproductive organs
- Extrinsic factors: environmental factors that influence life history strategies. For example, specific environmental factors might increase mortality or survivorship at a specific life stage.
- Fecundity: the ability to produce an abundance of offspring; the possible number of offspring that can be produced over a lifetime
- Fertility: the actual number of offspring produced by an individual or population
- Intersexual selection: mate choice, typically by females, based on desirable characteristics (ex: peacocks tail)
- Intrasexual selection: competition within one gender to be able to mate (ex: males fighting over territory)
- Intrinsic factors: factors based on an individual’s genotype that influence life history strategies, including development, genetics, physiology
- Iteroparity: reproductive strategy in which an individual reproduces many times during their lifespan; from Latin itero-, meaning “to repeat”.
- K-Strategist: a species with a large body size and long life span that produces fewer offspring later in life. Offspring have a high survival rate and high level of parental investment; populations are limited by carrying capacity (K)
- Life History: a species’ typical lifetime pattern of growth, development, and reproduction. Takes into account:
- Age and size at reproduction
- Typical lifetime length
- Number of offspring produced over the course of an individual’s lifetime
- Mating Systems:
- Monogamy: one male, one female – largest time commitment
- Polygamy: A broad term that refers to any mating system in which an individual has more than one mate during a breeding season; in these mating systems, individuals often defend limited resources
- Polygyny: one male, multiple females
- Polyandry: multiple males, one female
- Polygynandry: both females and males mate with multiple partners
- Promiscuity: males and females have multiple mating partners in a breeding season; mating can appear random
- Monecious: plants with both male and female reproductive organs
- Precocial: offspring are born more developed and independent. Require very little to no parental care
- r-Strategist: a species with a small body size and short life span that produces lots of offspring earlier in life. Offspring have a low survival rate and low level of parental investment; populations are limited by the maximum reproductive capacity (r)
- Semelparity: reproductive strategy in which an individual has only one reproductive cycling during their lifespan. Some semelparous species produce many offspring all at once, at the same time as other individuals in their population (like cicadas). Others species invest so much of their energy in finding a mate and reproducing, that there’s no energy left to live (like salmon). And for some species (like praying mantis and some spiders), the female may consume the male after copulation. From Latin semel-, meaning “one time”.
- Sexual reproduction: produces genetically distinct offspring
Outline of Notes:
Life History Strategies:
- Life History: A species’ typical lifetime pattern of growth, development, and reproduction
- Takes into account age and size at reproduction, length of lifetime, and number of offspring produced over the course of an individual’s lifetime.
- An organism’s life history is informed by both intrinsic and extrinsic factors:
- Extrinsic factors are based on the environment:
- Age-specific mortality
- Survivorship
- Extrinsic factors are based on the environment:
-
- Intrinsic factors are based on an individual’s genotype:
- Time of reproduction in life
- Number of offspring
- Asexual or sexual reproduction
- Timing/season of reproduction
- Intrinsic factors are based on an individual’s genotype:
Question from a Student:
“Can anyone help me understand why this is considered intrinsic? I understand that animals follow seasonal reproduction timelines, but the climate’s affect on reproduction seems like an extrinsic factor.”
Answer: Great question! I tend to consider reproductive strategies to include traits that are inherent to a particular species (i.e., life history strategies as one of many traits that have evolved differently across species). However, you’re absolutely correct that extrinsic factors like temperature could also affect the timing of reproduction.
For example, in plants seasonality can be considered in relation to temperature (which fluctuates), and also the length of the day as it relates to light (i.e., photoperiod, which is specific to a season and much less prone to change). Another thing to consider with regards to timing is that many plants have developed the means to stop themselves from “selfing” (i.e., self-fertilization), by extending male and female parts at separate or distinct times – a process which does inherently chronologically lock the timing and viability of their reproduction.
Species must make trade-offs in order to maximize the return on energetic investments. Trade-offs are inherent to different life histories:
-
- Reproduce early in life, or delayed?
- Many small offspring, or few and larger?
- Asexual or Sexual reproduction?
- Timing/season of reproduction?
- Animals can be classified into two categories, based on their life histories:
1. r-selected species: Tend to have shorter lives, have smaller bodies, and many offspring
-
- Reach carrying capacity quickly but have large amplitudes and variation around carrying capacity- boom and bust cycle
2. K-selected species: Tend to have longer lives, larger bodies, and fewer offspring
-
- Reach carrying capacity slower and have shallower amplitudes of population stability at carrying capacity
→ The R-K spectrum is often a generalization and cannot account for all of the factors different species face.
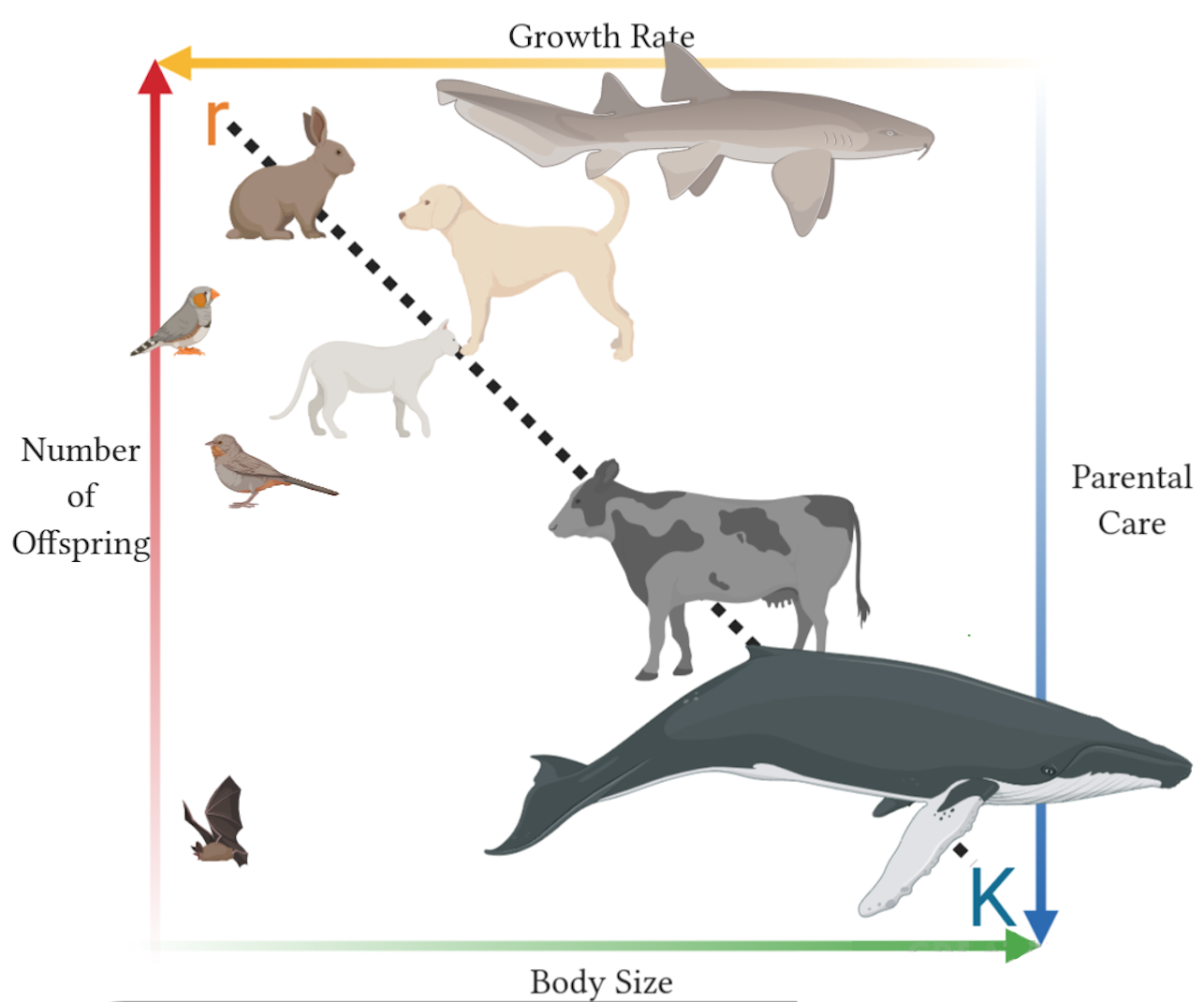
Asexual Reproduction Genetically identical offspring (though, note that random mutations can still occur in asexual reproduction!) |
Sexual Reproduction Genetically distinct offspring |
+ Only requires one individual
+ All individuals are able to reproduce + DNA is adapted to environment + Reduced energy expenditure – Loss in genetic variation |
+ Much more genetic variation (broader range of potential responses to a changing environment)
– Energy intensive (ex: gonad development, migration to spawning grounds, competition for mates, parental care and nesting) – Individuals only pass half of their genes to subsequent generations |
- Some species can change sex in response to:
- Changes in population’s sex ratio
- Availability of resources
- Energy cost of producing genetic material
Energy Investment and Mating Systems
- Sexual reproduction is energy intensive, especially for females: reproductive effort
- Gonad development
- Movement to spawning area
- Competition for mates
- Nesting
- Parental care
- Females need to balance the expense of growth versus offspring production
-
- For example, female Sardinella fish can either grow faster or produce more eggs (Figure 2).
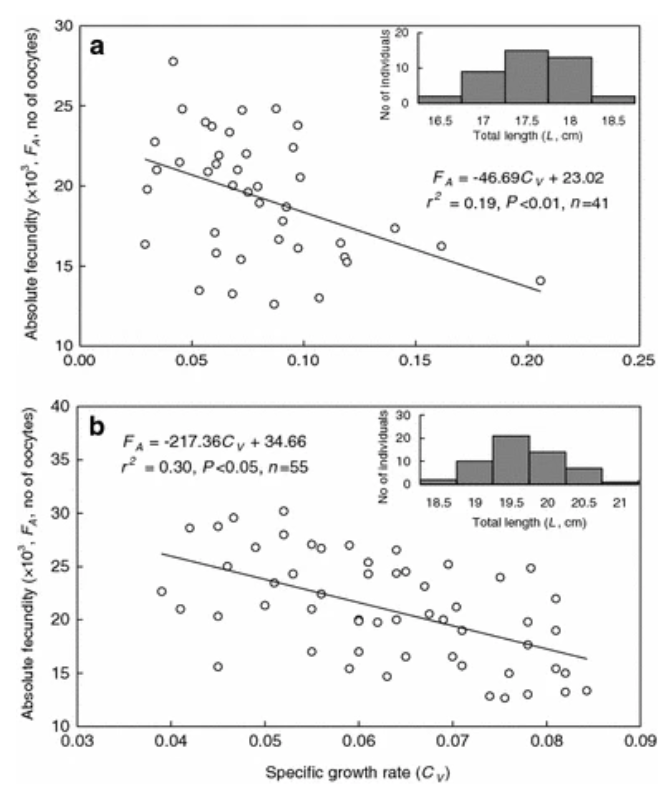
2-year-old females are generally smaller in comparison to females at three years old, who have a longer body length.
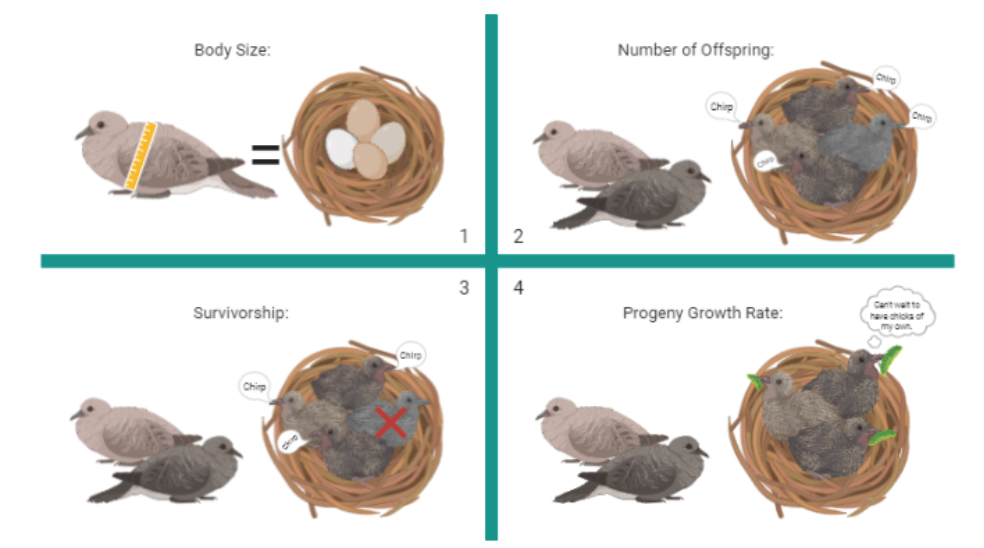
- Early investment to support reproduction later in life could cost you in the future:
- Mortality rate increases dramatically after having offspring
- Fecundity decreases with age and with reproduction
- Optimal allocation to reproduction maximizes parent survival:
- Often at intermediate reproductive effort
- Maximized total reproductive success over a lifetime
- Approaches to allocation:
- Semelparous: Many offspring per reproductive event, low care, low survival rate
- Usually precocial young
- Iteroparous: Few offspring per reproductive event, high care, high survival rate
- Usually altricial young
- Semelparous: Many offspring per reproductive event, low care, low survival rate
- Species engage in different mating systems, often depending on varying reproductive needs.
- Monogamy: one male/female pair, often with long time commitment
- Polygamy: multiple individuals within a system, competition over limited resources ensues
- Types of Polygamy:
- Polygyny: one male/multiple females
- Polyandry: multiple males/one female
- Promiscuity: multiple males/multiple females
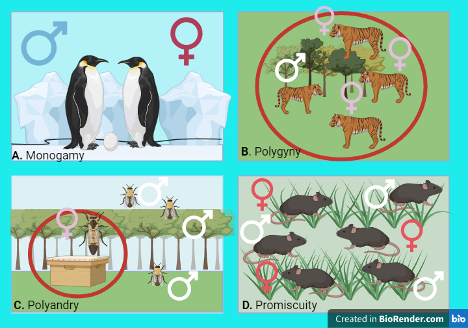
- Sexual selection can depend on:
- Morphology
- Behavior
- Social status
- Desirable territory
Intrasexual Selection: |
Intersexual Selection: |
Competition between individuals of the same sex (generally males) to control access to mates |
One sex (generally females) selects mates based on desirable traits, which are often displayed through courtship. |
- Desired characteristics are usually indicators of good fitness. Examples include:
- Plumage:
- Color: Indicates that a potential mate forages effectively for high-quality foods, and has enough surplus energy and nutrients (especially vitamins) to produce brightly colored feathers.
- Length: Indicates that the bird is able to evade predation even with long feathers that inhibit movement.
- Song complexity: Can indicate a longer lifespan as many songs are learned behaviors
- Plumage:
- Note, however, that some traits that are desirable for mating, actually impair an individual’s chances of survival (e.g., male peacock tail). These exaggerated traits are a result of runaway selection, which occurs when a secondary sexual trait expressed in one sex becomes genetically correlated with a preference for the trait in the other sex, resulting in a positive feedback loop.
Plant Life History Strategies:
- Three main factors are theorized to contribute to plant life history strategies:
-
- Competition
- Disturbance: High levels select for high growth rate and large number of offspring
- Stress: High levels select for large size and more parental care
→ Different combinations of these factors favor different life history strategies.
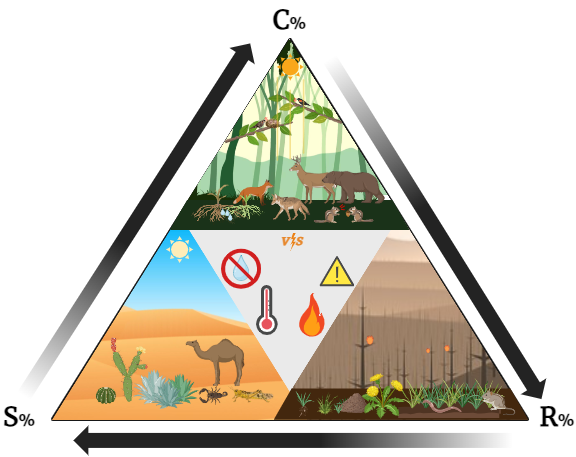
- Low stress, low disturbance: favors competitive species
- Fast growth rate, low energy investment into producing seeds and “parental care”
- Low stress, high disturbance: favors ruderal species
- Small size at maturity, fast growth rate, large number of offspring, low “parental care”
- High stress, low disturbance: favors stress-tolerant species
- Large size at maturity, low growth rate, small seed bank, high “parental care”
- Plant version of parental care:
- Seed size
- Secondary compounds (tannins, toxification of environment)
- Plants can be either dioecious or monecious:
- Dioecious plants: have either male or female reproductive organs
- Monecious plants: have both male and female reproductive organs
- Many monecious plants have evolved strategies to avoid self-pollination because it lowers the fitness of their offspring. (See Question from a Student above.)
Key Takeaways
Overarching Themes and Unifying Concepts:
- Species evolve different life history strategies in response to different environmental conditions
- Fecundity can be a great determinant of an individual species’ life history
- Proper trade-offs and resource allocation are crucial for survival
- r-selected invest in a high number of offspring with low individual survivorship, resulting in “boom and bust” population dynamics.
- K-selected species invest in more parental care (and therefore greater survivorship) for relatively fewer offspring, resulting in more stable populations.
- Asexual vs sexual reproduction:
- Each has its own unique costs and benefits
- Sexual reproduction takes many forms, and different environments favor different mating systems.
- Multiple approaches to offspring:
- Iteroparity vs Semelparity
- Precocial vs altricial young
- Iteroparity vs Semelparity
- Mates are selected for a variety of reasons
- Often with the best chance of reproductive success in mind
- Sexual reproduction is costly, especially to females
- Eggs/seeds are larger and less numerous than sperm/pollen
- Female mortality rate increases after breeding
- In many species, females (may be more likely than males to) invest additional energy in parental care after offspring hatch / are born.
How might this information be applied to address grand challenges?
- The life history of an endangered species could be studied to see if there is a way to improve their chances of survival
- Find the most successful life history for pollutant-mitigating vegetation to ensure their survival
- Sexual selection helps determine healthy individuals of a species
Unsolved Mysteries and Future Research:
- Are there other life histories or reproductive strategies that are currently unknown to the field of ecology?
- What impact will our decreasing childbirth rate have on the life history of humans?
- How do life histories evolve in response to a natural disaster?
- What kinds of intrinsic relationships are there between species’ mating systems and their greater social systems?
Blog Style Summary:
Ever wondered how the characteristics that make you, you (and bugs, bugs; and wolves, wolves; and fish, fish; etc.) came to be? What I mean by this is, have you ever wondered why you are succeeding in life? Let me back up for a second. When I say succeed, I mean your fitness level, or your ability to survive in the world and reproduce. Organisms have an inherent set of life history strategies, or factors that allow them to go about living life (and succeeding). Each species has different strategies from the next, depending on factors such as their genetics and environment. For instance, let’s take a quick look at body size. When you’re walking down the street, you don’t see a bird the size of a human or a dog the size of a lizard, right? It would be really weird if you did… because different species have an optimal body size that they grow to (on the norm). A songbird doesn’t need to be the size of a human because it’s just fine being small. Its size is optimal for its energy intake and output, what it eats, and how it goes about living life.
Well, life histories are all about trade-offs, as you’ll see throughout this blog. Remember when we mentioned you don’t see a bird the size of a human? Well, it turns out they actually do exist. Meet the Harpy eagle.
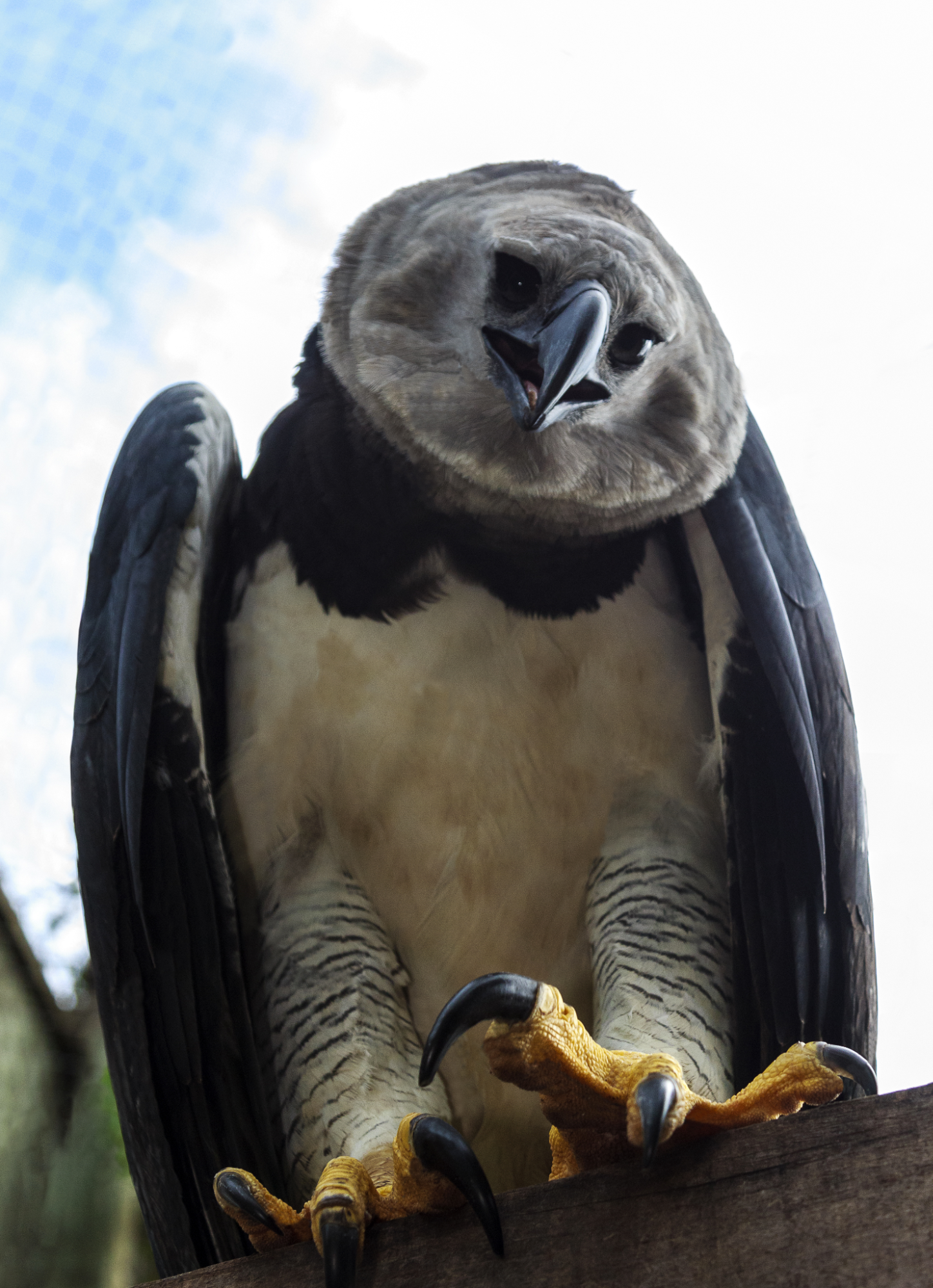
Crazy, right? This bird is huge, weighing between 9-11 pounds. So naturally they require a LOT of energy intake for their body size. Harpies eat sloths, monkeys, and opossums, which can take a significant amount of energy to grab and lift. So, as a trade-off to conserve energy these birds are rarely seen flying about over the forest canopies where they live (which is generally in South America). They rarely fly long distances, and spend most of their time perched, waiting for meals to cross their path (“Largest Eagles in the World Are so Big That Their Talons Are Bigger than Bear Claws,” 2021). They also lay two eggs every 2-4 years, but ignore the second egg once the first one hatches. They focus all of their attention on protecting and raising their chick for about 6-7 months (Harpy Eagle Facts, 2011). As I said earlier, these birds require a lot of energy, and it’s all about supply and demand, so the parents focus their extra energy on the survival of only one fledgling.
Let’s talk some more about parental care; you know, the way your parents raise you. Believe it or not, some species of animals get kicked out of the house immediately (unlike that guy you know that’s 25 and still mooching off of his parents). Some species put more time and care into raising their young, and because of that have fewer offspring, like humans and elephants. Other animals have many offspring at once in the hopes that one or a few might make it, like rabbits.
A lot of that is based on whether you are a precocial or altricial species (there’s also semi-altricial and semi-precocial). The offspring of precocial species develop quickly and are capable of almost immediately surviving on their own. Altricial offspring are born helpless and develop much slower. To illustrate this point, below is an image of two different bird species’ hatchlings, with two different life history strategies. The chicks on the left (purple martins) are naked, blind, and helpless when first hatched, while the chick on the right (a killdeer) is feathered, fully sighted, and mobile upon hatching.
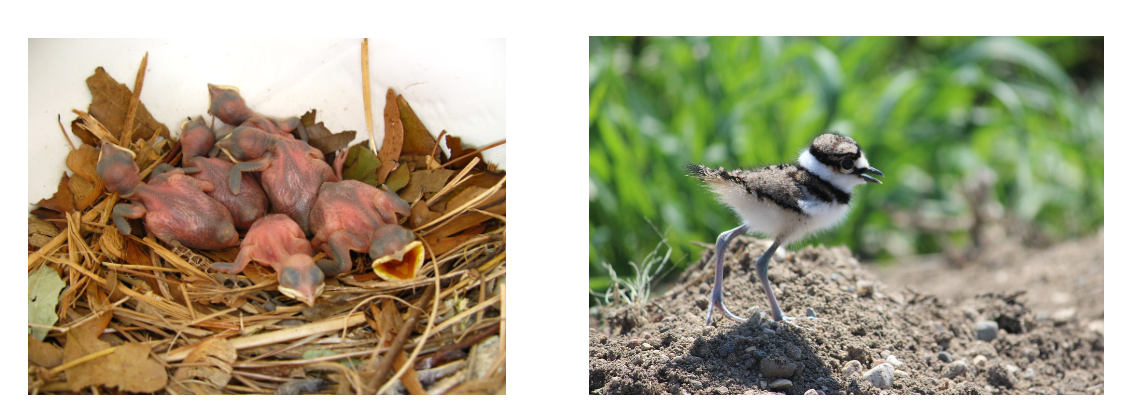
Personally, I’d much rather have a baby that didn’t whine and cry every time it needed something. So at first glance, I wonder what benefit a species would gain from having their young born basically helpless? Well, let’s not forget that life history strategies are all about trade-offs. Generally, the altricial species spend their time gathering sufficient food for their helpless offspring, while the females of precocial species have to eat twice as many calories as the altricial species to ensure they produce energy-rich eggs. Precocial animals are often born with big brains, but their adult brain is small in relation to their body size. On the other hand, altricial young are born small-brained but have significant postnatal brain growth and end up with proportionally larger brains as adults than precocial species (Precocial and Altricial Young, 1984).
Of course, you can’t have life without reproduction, and there are many trade-offs in this area too. First, there is the choice between asexual and sexual reproduction. Asexual reproduction is more energy efficient and only requires one organism, but reduces genetic variation as all offspring produced are genetically identical. Sexual reproduction is more energy intensive but results in more genetic variation, which can result in better fitness. This extra effort disproportionately falls on female members of species, as they are the ones producing eggs/milk.
It may seem like this is not actually a choice as we cannot just switch between these two options. However, many plants can do just this through a process called vegetative propagation. Almost all plants retain totipotent cells–those with the ability to grow into many different cells–throughout their life, allowing for asexual reproduction to be possible. You have probably seen many examples of vegetative propagation in your life. For example, have you ever left a potato too long in your fridge and seen many shoots coming out of it? Since potatoes are not the plant’s fruit, this cannot be sexual reproduction. In fact, these are fully-fledged, genetically identical plants growing from a modified root. Aren’t plants neat?
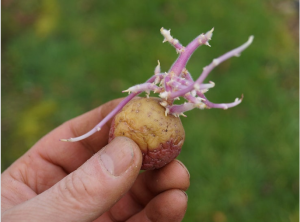
How can we summarize these different approaches? Well, living things generally follow two life history strategies. r-strategists have lots of offspring but do not put much effort into raising them. K-strategists, on the other hand, delay having offspring and have fewer offspring, but provide more parental care. You can see how these overall strategies mesh with more specific life history traits. r-strategists would benefit from precocial offspring, as they can survive without much parental care. Of course, we humans are the ultimate K-strategists and our babies are pretty useless. I don’t think any other animal cares for its offspring for 18+ years.
Life history traits are important as they are key to understanding how and why a species’ population has and will change. As we humans drive more and more species towards extinction, life history will help us understand which species are the most vulnerable and how to best preserve our planet.
Spotlight on NC:
The Carolina gopher frog is an endangered resident of North Carolina’s coastal plains and sandhills [1]. Gopher frogs (Rana capito) are a member of the “true frog” family, Ranidae, even though their dark, bumpy skin makes them look a lot like a toad. Their native range coincides with the historic distribution of longleaf pine savannas across the southeastern United States, from North Carolina to western Florida [2]; However, widespread deforestation (up to 98%) of longleaf pine forests [3] has resulted in severe habitat fragmentation and thus isolated gopher frog populations.
The gopher frog is a specialist with strict habitat requirements; they spend most of their life in burrows, but emerge yearly to breed in wetlands. Gopher frogs are considered iteroparous because they can reproduce multiple times throughout their lifetime. Due to their independent, underground lifestyle, not much is known about the life history of gopher frogs besides their breeding biology. For example, data on gopher frog longevity is incomplete, but one gopher frog is known to have lived 9 years in captivity [4]. In North Carolina, gopher frogs’ dens are often found in the stump holes of fallen pines [5]; In their more southern range, gopher frogs are a commensal species, relying heavily on the burrows of gopher tortoises for refuge [6]. To mate, gopher frogs require access to nearby wetland habitats containing grassy vegetation and an open forest canopy [7]. Gopher frogs in North Carolina have been observed to travel up to 3.5 km from their den site to access suitable breeding ponds [5]. Only 7 populations of gopher frogs remain across the state of North Carolina (Figure 1).
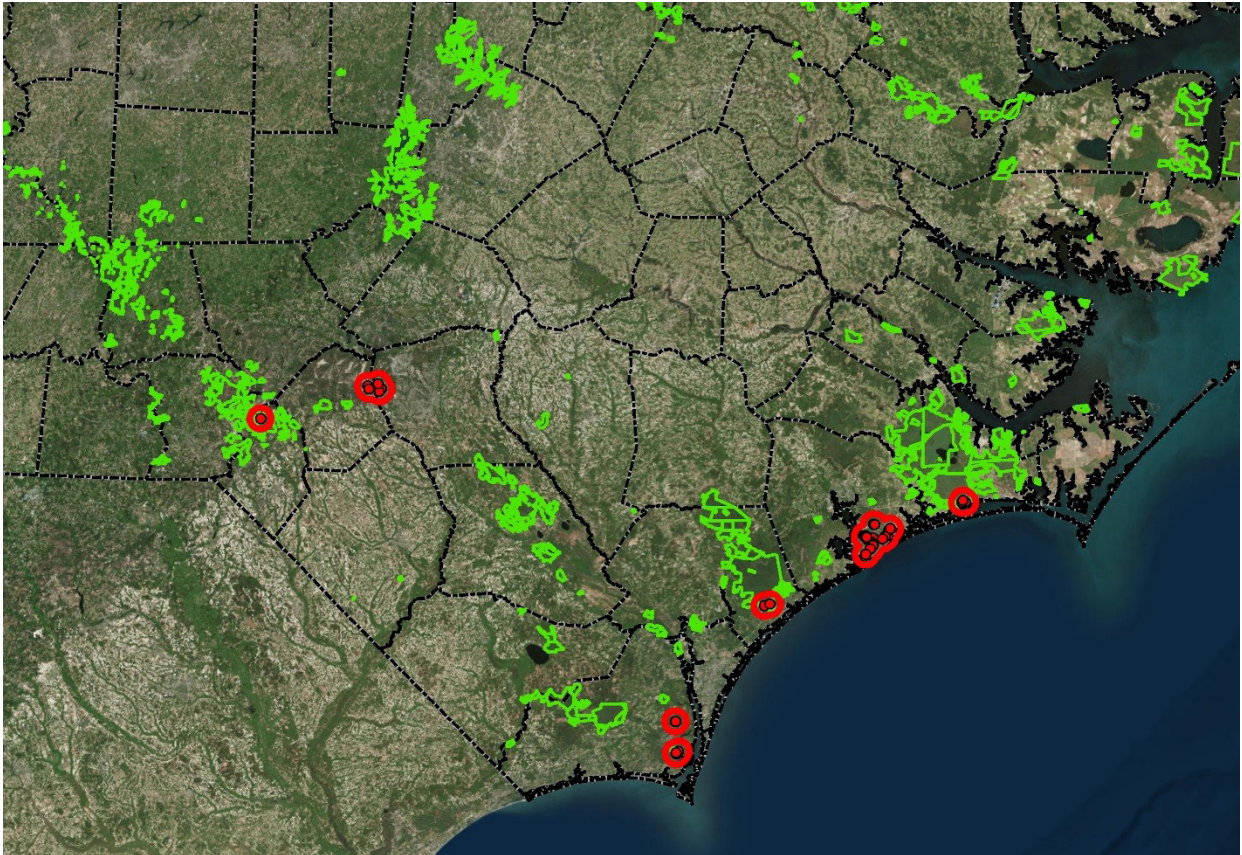
The Breeding season for Carolina gopher frogs lasts from January to April, with mating occurring immediately after a heavy rain event. They select ephemeral (temporary) ponds in the coastal plain which are absent of fish. This means their main predators during the breeding season are caddisfly and dragonfly larvae, diving beetles, and turtles. The males stay in these ephemeral ponds for around a month, calling for females. The female frogs stay for less than a week, usually laying one cluster of thousands of eggs, which are then externally fertilized by the males. This means the Carolina gopher frog is polygynous! It may surprise some that the larval stage for this species ranges from 87 to 225 days and they reach sexual maturity at 1.5-2 years old, but these are relatively typical timelines for a lot of frogs [8]. Furthermore, like most other amphibians, these frogs’ reproductive strategy is r-selection instead of K-selection. This means gopher frogs invest more energy into producing a high quantity of offspring versus high-quality offspring that they have to take care of for a long time. This concept is demonstrated in Figure 9 below.
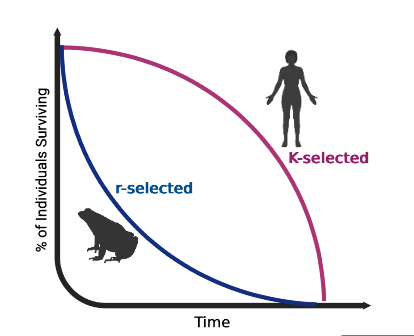
There are a number of ecological challenges that gopher frogs face. If you are familiar with the idea of the “Sixth Mass Extinction” you’ll know that amphibians are some of the most at-risk species. One of the ecological factors that is affecting gopher frogs in particular is the deforestation of their habitat. The longleaf pine forests that gopher frogs call home are in fact endangered themselves. Longleaf pine forests have been heavily deforested and have a difficult time recovering because they are dependent on seasonal burns for reproduction. The reduction in this habitat has affected the gopher frog population in NC. The government is taking some steps to address the ecological challenges that gopher frogs are facing: the Department of Defense is implementing new strategies for conservation for bases that include gopher frogs and their habitat [9]. In 2022, the Biden administration reinstated the “Endangered” status to gopher frogs, overturning a decision made by the previous administration. This will allow more of the gopher frog’s natural habitat to be protected for conservation [10]. The North Carolina Zoo also has a gopher frog breeding and release program led by Dustin Smith, which works to protect gopher frog habitat as they release and monitor populations.
Habitat fragmentation is one factor that has been contributing to the species’ decline. The construction of roads, towns, and cities have directly destroyed their habitat but has also reduced gene flow between subpopulations. As roads are built through longleaf pine forests they cut through frog habitats, creating a barrier separating populations. When mating season comes, frogs will either have to risk crossing the road and potentially dying, or they must mate with those closest to them. This leads to reduced genetic diversity over generations. Another ecological challenge that gopher frogs are facing is the intensifying drought in the southeast United States (https://www.drought.gov/drought-status-updates/drought-status-update-southeast-us). Drought can disrupt the reproduction cycle and development of gopher frogs in such a way that reduces their populations, as they rely on the ephemeral pools produced by heavy rainfall [8].
Due to the fact that habitat loss is the main challenge faced by the species, a large amount of future conservation efforts rely on the conservation and restoration of longleaf pine forests. One of the main reasons that longleaf pine forests have been in decline for the past century is due to the fact that they require frequent, low-intensity fires [11]. For much of the 20th century, the US government has had an extreme anti-wildfire campaign [12]. Forest managers believed that all forest fires were dangerous or bad for the health and growth of forests. However, longleaf pines require fire to eliminate competitors and allow their saplings to grow [11]. Nutrient-poor soils are also common in this ecosystem [12], meaning that longleaf and many of the other plant species the gopher frog relies on are considered stress-tolerant. The suppression of the fires, along with logging and habitat loss due to development, are the reasons that this ecosystem has been on the decline for so long [12]. In order for species like the gopher frog to have a chance at recovery, proper management of the remaining longleaf pine forest, along with restoration efforts to return longleaf pines to their native range, are required. The NC Wildlife Resource Commission has strategized that the best course of action for the conservation and recovery of gopher frogs is to use prescribed fire to create more breeding habitats [4]. Essentially, if there is more habitat, wildlife managers can attempt to establish new populations. Thankfully, there are many organizations working to conserve and restore longleaf pine forests across the southeast. Organizations like the Longleaf Alliance have listed the recovery of the gopher frog (among other wildlife species) as one of the main benefits of supporting longleaf restoration. If we hope to better understand the interesting life history of the gopher frog, it is important that we work to conserve the species for future generations to study.
How to Apply Ecology in a Changing World
Life History refers to the way any given organism reproduces, grows, and behaves throughout its life cycle. Different tactics are employed by different species to optimize fecundity over an individual’s lifespan (Bednekoff, 2019). We humans put a lot of care and energy into relatively few offspring to ensure their survival. Other animals, like leopard frogs, lay a massive clutch of eggs and then leave them, relying on the large number of offspring they’ve created to allow for at least a couple to mature and reproduce without any parental care. A sand tiger shark, however, gives birth to only one offspring at a time, like a human; but because its pup is precocial (already mostly adept and mature at the time of birth), it provides no parental guidance, like a leopard frog. There are countless unique life history strategies that can be observed, and animals differ widely from species to species, but many share similar characteristics. The mode of reproduction, general mating habits, relative maturity of the young when they are born, migration patterns, and other traits are often common between more than one species. Distinct adaptations that help organisms survive in different habitats and at different times are what make each strategy effective. Specific life histories often intrinsically involve a certain ecosystem or habitat. A great example is the importance of estuaries and salt marshes to certain species here in North Carolina.
The coast of the United States from North Carolina to Florida is home to approximately one million acres of salt marsh habitat, also called estuaries, wetlands, pocosins, swamps, or bogs; these areas are rich in species diversity. This living coastline is an important part of many species’ life histories, and it also provides ecosystem services for us humans, acting as a natural filtration system for our inland freshwater networks. Salt marshes also serve to store water, prevent erosion and regulate flooding (South Atlantic, 2023).
Any ecosystem is at risk of adverse disturbances, but the estuarine habitats in North Carolina are especially sensitive. Pollution, urban development, and the introduction of non-native species are all caused by humans; but natural disturbances like wind, tidal currents, deep freezes, and waves can also affect the life histories of species in the ecosystem. There natural events may occur frequently, like the tides which result in a constant flux of salinity, which native species have adapted to survive (US Department of Commerce, 2019). Irregular disturbances like storms or an increase of nutrients are harder for species to adapt to, and humans can destroy an entire ecosystem by building dams, draining, and dredging. Changes to the estuarine ecosystem can have large and lasting effects on the survival of species that depend on it to complete their life cycle.
One such species, the striped bass (Morone saxatilis), exhibits an interesting life history strategy known as anadromy. Anadromous fish are born in freshwater, grow and mature in marine environments, but return to freshwater environments to spawn. Anadromous fish migrations are responsible for some incredible ecological spectacles: thousands of large striped bass ascend rivers in the spring, usually from April to June, and spawn at the surface in a shallow river. The fertilized eggs will drift downstream and eventually hatch. The larval fish will grow in estuarine environments, and at a larger size they will move to the open ocean where they will continue to grow until they mature and begin ascending freshwater rivers to spawn themselves (see Figure 10). Intact and healthy estuarine habitats are critical to the success of striped bass populations (Fisheries, 2023).

Another creature that depends on wetlands for its life cycle is a bird called the clapper rail. Every part of the life history of clapper rails is tied to intact salt marsh habitat. They need it for protection, feeding, and for successful reproduction as well as growth of offspring. The rails breed and nest in the spring, when they utilize different marsh plants to build and disguise their nests. Their eggs incubate for just over two weeks. When they hatch, the chicks are precocial (already mostly developed); within days they will be capable of gathering their own food, and within weeks they will be able to fly. The young birds utilize the tall marsh grasses for cover while they forage in shallow water and mud (Fuller, 2011). Both the clapper rail and striped bass are native to North Carolina, and their life history strategies evolved in estuarine ecosystems like the ones we have on our coast.
We need to approach saving Southeastern salt marsh habitats from multiple perspectives. We need to have people on the ground, surveying the damage, clearing invasive species and planting native ones, and removing garbage, toxic waste, and pollution. We need lobbyists and legislators working to change policies and regulations to increase protection for pocosins and ensure their preservation; and we need still others working in public outreach to educate people about the importance of saving this ecosystem. There are many initiatives that are doing just that.
In North Carolina alone, there are at least 10 restoration projects in place. Carolina Beach State Park recently completed a $1.9 million rehabilitation project to restore 10 acres along the Cape Fear River Basin. There are two projects currently being led by the North Carolina Coastal Federation (Fisheries, 2023). These examples are mostly focused on the hands-on element of restoration, actually getting out there and cleaning, clearing, and planting, which is an effective way to conserve the ecosystem. But other projects expand their focus to include changing policies and public outreach.
The South Atlantic Salt Marsh Initiative (SASMI) is a 10-year plan that began in May 2023. This initiative partners with federal, state, and military agencies, property-buying agencies, state and local parks and private landowners to more effectively manage this resource. SASMI is working in changing and enforcing policies, monetarily supporting hands-on restoration projects focused on protecting, restoring and conserving the pocosins, and working to educate and engage the public (South Atlantic, 2023). In ten years, they hope to not see any overall loss in benefits these wetlands provide to fish, wildlife or people.
If we approach saving North Carolina wetlands with an interdisciplinary mindset, then we have a better chance at saving our native species that have evolved with salt marshes and have life history traits perfectly suited to this ecosystem and would not survive anywhere else.
Featured Ecologists
Dr. Cathy Bodinof Jachowski, MS, PhD
Dr. Cathy Bodinof Jachowski studies how freshwater aquatic species interact with their environments and how they will respond to environmental change. Jachowski primarily works with hellbenders but has also studied mussels, alligators, and turtles. She has closely examined how the life history of hellbenders will affect the habitat they use. Her work has shown what types of habitat are important for juvenile hellbenders, and how to increase adult hellbenders’ use of artificial nest boxes. From these studies come important conservation implications for hellbenders. To have a healthy population of hellbenders, a stream needs to have diverse rock sizes (and therefore diverse habitats) to provide hiding places for juveniles and adults. Anthropogenic changes, like sedimentation, can negatively impact the amount of habitat available for hellbenders. When streams don’t have places for adult hellbenders to hide and nest, artificial nest boxes can be a valuable tool. Additionally, Jachowski has investigated how captive-reared hellbenders fare once released into the wild. Her studies provide evidence that release may be a viable way to supplement endangered wild populations, given that released hellbenders had similar survival rates to wild hellbenders. The main purpose of Jachowski’s research is to gather information that will support development of the best conservation strategies for freshwater animals.
Student contributors:
Note Outline: Adrian Chamberlin, Noel Griffin, James Holmes, Emily Usinger, Shelby Williford
Blog Style Summary: Kimmy Gebbia, Madison Allen, Landon Wang
Spotlight on NC: Ian Warr, Kelly Koehler, Jackson Wimpy, Sophie Meng
How to Apply Ecology in a Changing World: H Attix, Grace Gooding, Sean Sullivan
Featured Ecologists: Abi Finch
the ability to produce an abundance of offspring; the possible number of offspring that can be produced over a lifetime
plants that have either male or female reproductive organs
plants with both male and female reproductive organs
one male, one female - largest time commitment
reproductive strategy in which an individual reproduces many times during their lifespan
reproductive strategy in which an individual has only one reproductive cycling during their lifespan
A species’ typical lifetime pattern of growth, development, and reproduction. Takes into account:
Age and size at reproduction
Typical lifetime length
Number of offspring produced over the course of an individual’s lifetime
offspring are born more developed and independent. Require very little to no parental care
offspring are born less developed, requiring significant amounts of parental care
produces genetically distinct offspring
produces genetically identical offspring
a species with a small body size and short life span that produces lots of offspring earlier in life. Offspring have a low survival rate and low level of parental investment
a species with a large body size and long life span that produces fewer offspring later in life. Offspring have a high survival rate and high level of parental investment
A species’ typical lifetime pattern of growth, development, and reproduction. Takes into account:
Age and size at reproduction
Typical lifetime length
Number of offspring produced over the course of an individual’s lifetime
mating system in which one male mates with multiple females