Chapter 11: Ecosystem Ecology
Vocabulary:
- Assimilation: moving nutrients into cells where they are used to perform bodily functions
- Autotrophs: organisms that produce organic compounds via carbon transformation
- Chemotrophs: organisms that obtain energy through the oxidation of electron donating molecules in the environment
- Endothermic: a reaction that absorbs energy/heat into the system
- Enthalpy: amount of internal energy (heat) in a system
- Entropy: a measure of disorder, or how evenly energy is distributed in a system
- Exothermic: a reaction that releases heat into the surroundings (the system loses energy)
- Gross primary productivity (GPP): the total amount of carbon “fixed” (converted to organic compounds such as sugars) by producers during photosynthesis
- Kinetic energy: energy in motion, performing work at expense of potential energy
- Net primary production (NPP): amount of organic matter created from photosynthesis, expressed in units of organic matter per unit area per unit time
- NPP = (Gross primary productivity) – (Respiration by autotrophs)
- Potential energy: stored energy
- Secondary production: growth of new tissues and reproduction
- Transpiration: water that is lost from leaves into the surrounding air
Tip from a Student:
Enthalpy and entropy are similar in how they sound so I used to mix them up. A way I remember the difference is by the associating the “H” in enthalpy with “heat”, and “R” in entropy with “random”.
– Brandon Middlebrough
Outline of Notes:
Ecosystem Ecology:
- Food webs connect community ecology and ecosystem ecology.
- Ecosystem ecology centers around the transfer of energy and groups species by function
- Ex: Predators, primary consumers, primary producers, etc.
- To understand ecosystem ecology, you must first understand the Laws of Thermodynamics:
-
- First Law: Energy cannot be created or destroyed
-
-
- Chemical reactions can be:
- Endothermic: must absorb energy to complete the reaction
- Exothermic: releases energy into the environment
- Chemical reactions can be:
-
-
- Second Law: Energy transformation and transfer is inefficient
-
-
- Part of the energy assumes a form that cannot pass any further (e.g., heat) , decreasing potential energy and increasing entropy in a system
- Potential energy: stored energy
- Entropy: a measure of disorder in a system. As entropy increases, less energy is available to do work.
- In closed systems, all energy will eventually be lost to entropy.
- Living systems are open systems that receive a constant input of energy (solar radiation) that counteracts entropy
- Part of the energy assumes a form that cannot pass any further (e.g., heat) , decreasing potential energy and increasing entropy in a system
-

The 1st Law of Thermodynamics in Ecology:
Net Primary Production:
- Net primary production (NPP): the rate of mass per unit area of organic matter (standing crop biomass) created from photosynthesis, expressed in units of organic matter per unit area per unit time (ex: grams of Carbon per square meter per year)
- NPP = (Gross primary productivity) – (Respiration by autotrophs)
- Productivity is the rate at which organic matter is created through photosynthesis
- Biomass is the amount of organic matter produced through photosynthesis
- Environmental variables such as water, temperature, and light limit NPP:
- Water, temperature, and light = climate
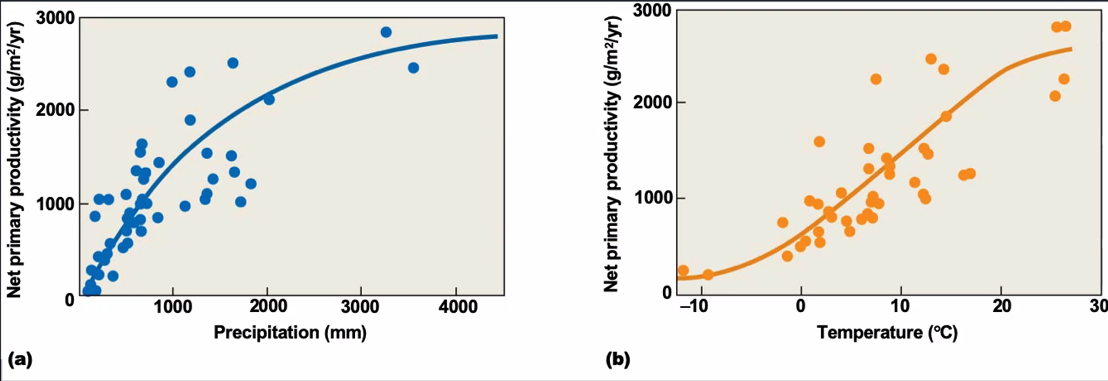
-
- NPP increases with increasing temperature and rainfall:
- Expected NPP is highest in ecosystems like tropical rainforests and lowest in ecosystems like frozen deserts
- Explanation: plant stomata will only stay open if they are able to replace water lost in transpiration. More rainfall = more water available for transpiration.
- Water availability limits both the rate of photosynthesis and the amount of leaves/surface area for transpiration.
- NPP increases with increasing temperature and rainfall:
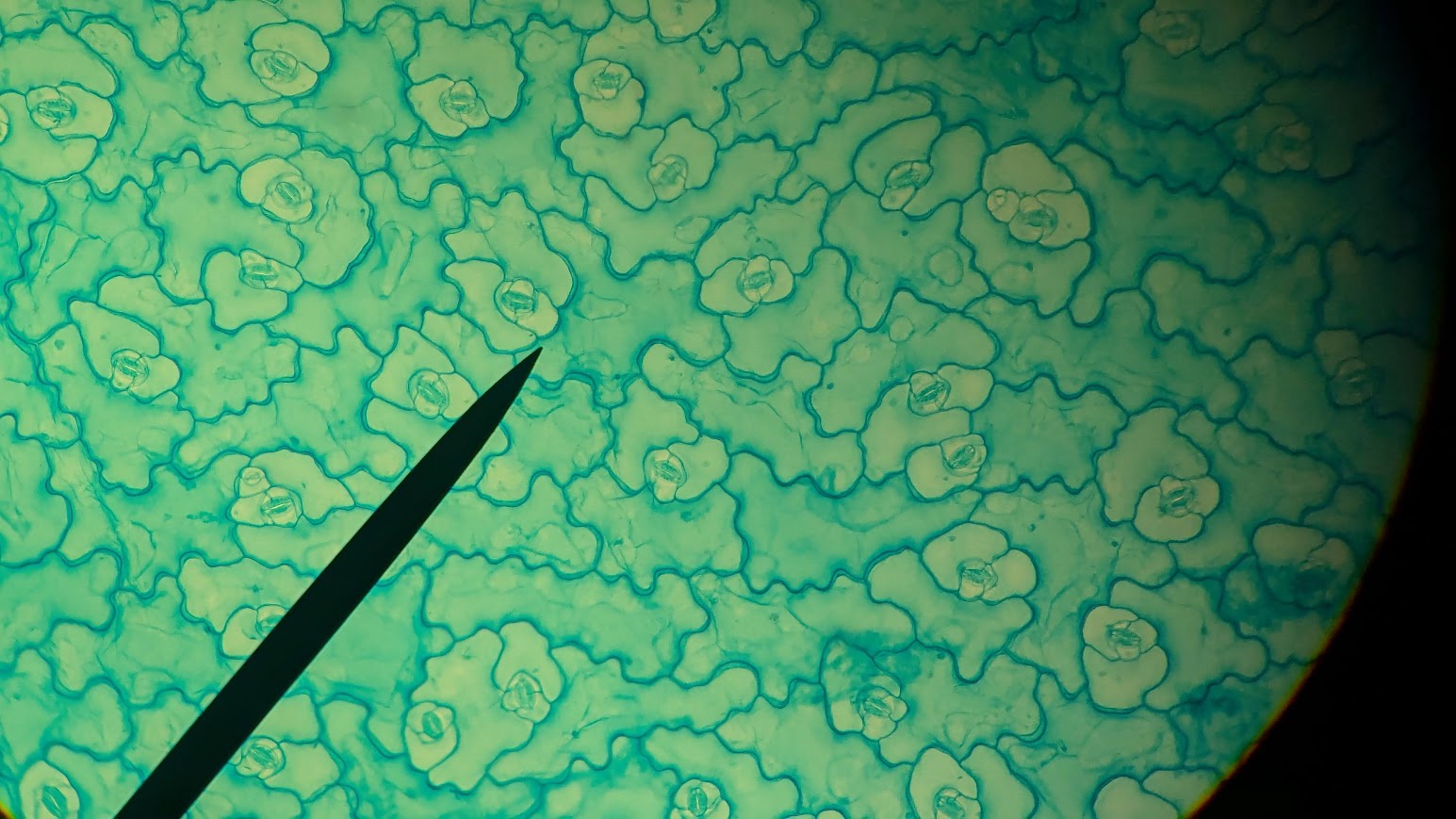
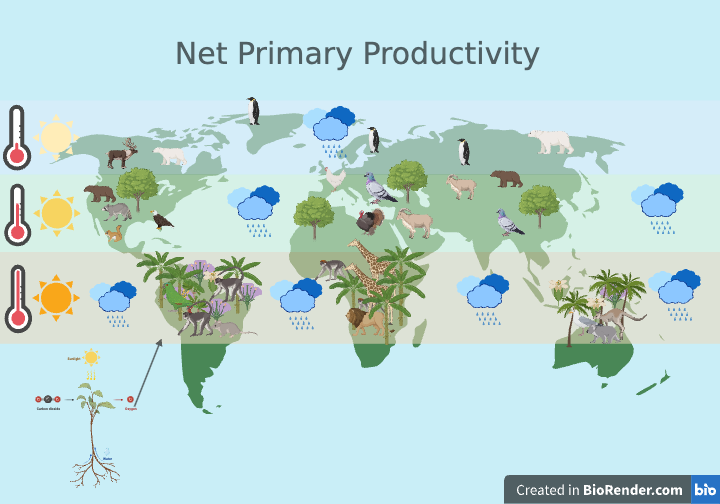
-
- NPP also varies with the length of the growing season:
- Growing season is largely a function of latitude
- In terrestrial ecosystems, seasonality of precipitation increases, growing season shortens, and net primary productivity decreases as we move away from the equator
- Highest NPP found near the equator as there is more sunlight year round
- NPP also varies with the length of the growing season:

Aquatic NPP:
- NPP is greatest in shallow waters → photosynthesis is limited to the photic zone
- Below the photic zone (>30m), many organisms rely on deadfalls or chemosynthesis
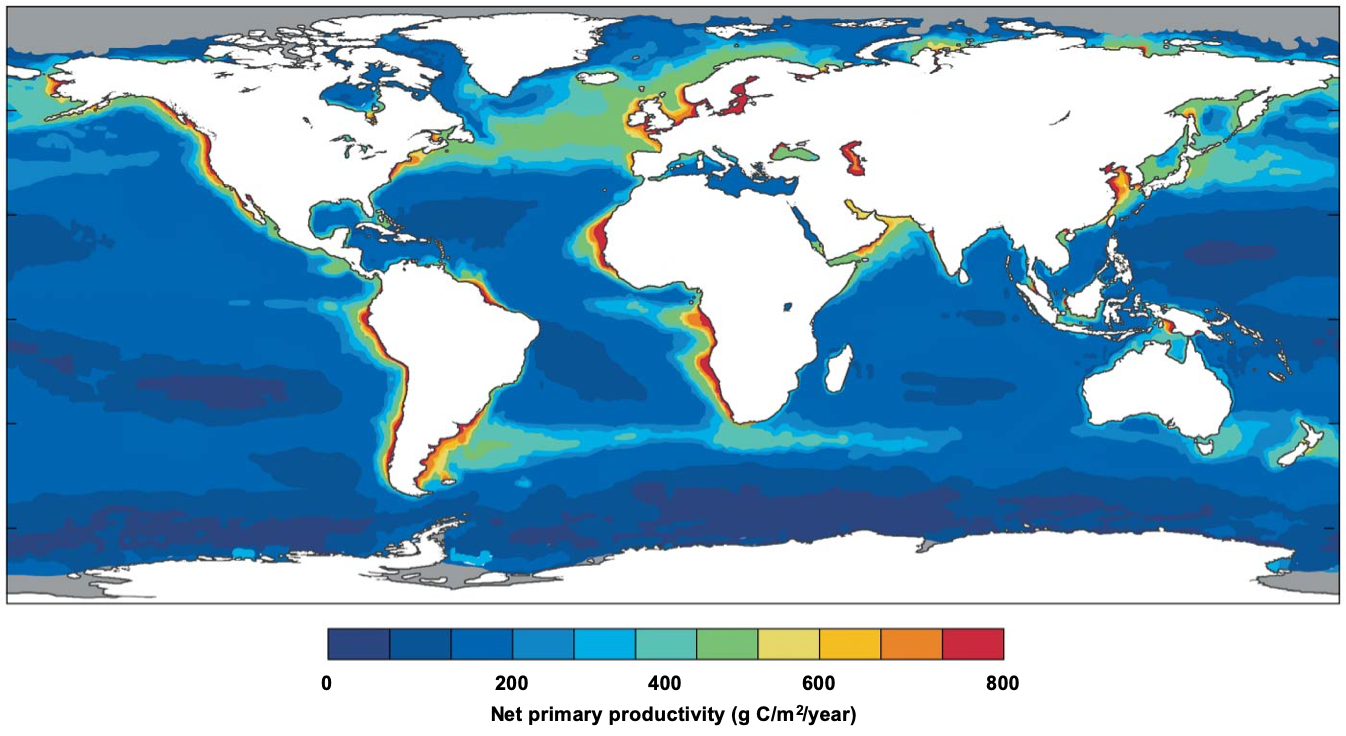
- Organisms such as krill migrate to the water surface during the day to feed on photosynthetic algae, then migrate back to the benthic layer at night.
- Krill transport nutrients to the benthic layer via defecation and by providing food to predators
- Vertical migrations such as this can be detected via acoustic sonar signals known as the deep scattering layer.
- Whale falls also bring nutrients from the surface to the benthic layer
- Krill transport nutrients to the benthic layer via defecation and by providing food to predators

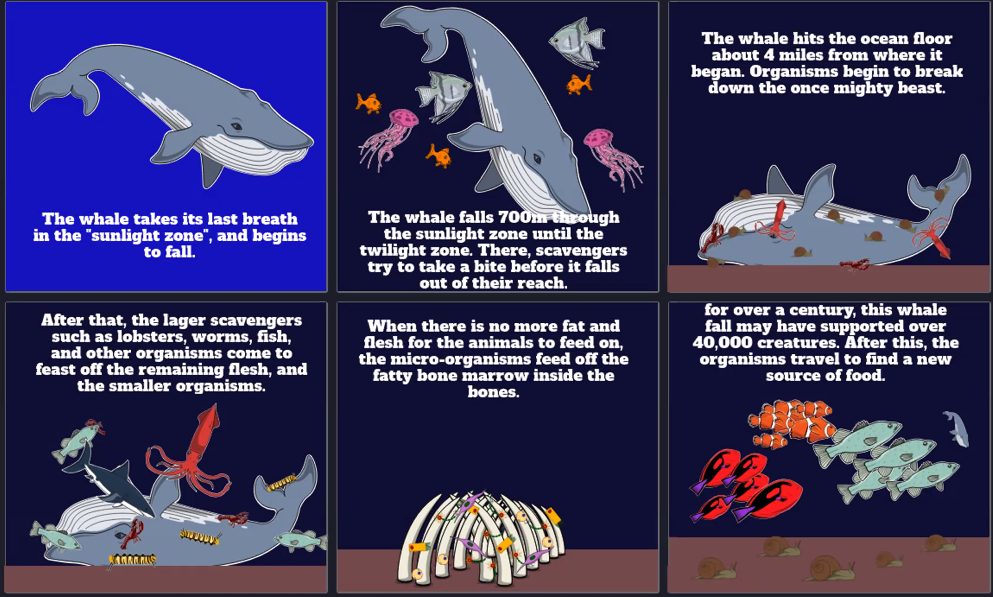
The 2nd Law of Thermodynamics in Ecology:
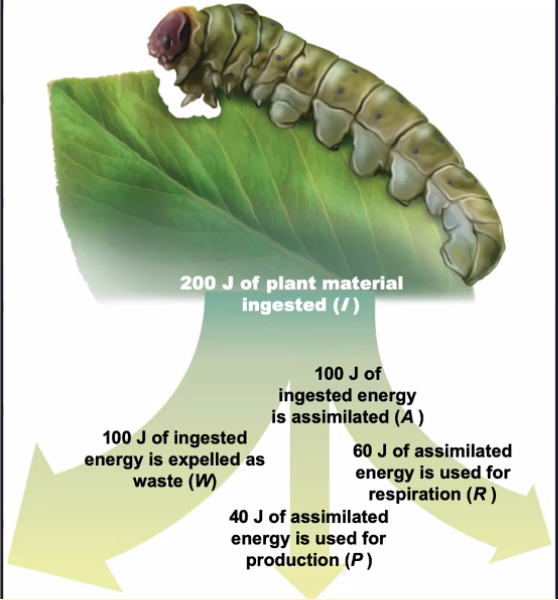
- Energy transformation is inefficient (entropy increases and enthalpy decreases)
- The constant input of solar energy fuels the ecosystem by increasing enthalpy. This counteracts entropy.
- Calculating relative efficiency of energy conversion:
- Assimilation efficiency = A/I
- Fraction of energy stored in body after expelling waste
- Production efficiency = P/A
- Fraction of usable assimilated energy after accounting for respiration
- Assimilation efficiency = A/I
- Secondary production: growth of new tissues and reproduction
- As NPP increases, we see:
- Overall increased biomass of herbivores, due to
- Increased herbivore body size (e.g., weight of individuals)
- Increased number of offspring (numerical response)
- Herbivores eating more (functional response)
- Overall increased biomass of herbivores, due to
- Ecosystems have two major food chains:
-
- Grazing: energy flow is unidirectional
-
-
- Source of energy: living plant biomass or NPP
-
-
- Detrital: energy flow is cyclical
-
-
- Source of energy: detritus (dead organic matter)
-
→ In both: energy is lost at each trophic level through respiration
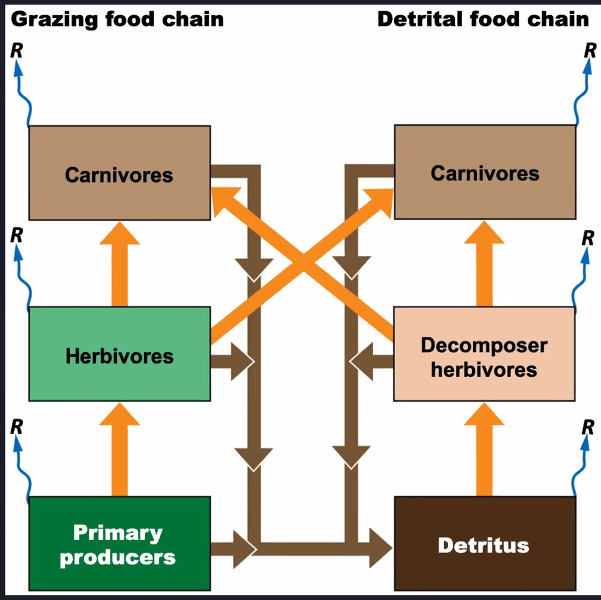
- The transfer of energy between trophic levels can be visualized through biomass pyramids:

-
- Only ~10% of energy gets transferred to the next trophic level
- Human impacts:
- Humans are estimated to use 3-40% of NPP directly, despite making up only 0.5% of the heterotrophic biomass on Earth
Key Takeaways
Overarching Themes and Unifying Concepts:
- Energy cannot be created or destroyed
- Energy transfer is inefficient- most energy is lost through respiration when being transferred between trophic levels
- Only ~10% of energy is transferred between trophic levels
- NPP increases with increasing water and temperature and varies with the length of the growing season.
- NPP is highest near the equator
- Ecosystems have two major food chains: Grazing and Detrital
How might this information be applied to address grand challenges?
- Ecosystem-based management has been a staple of Western science for decades and is particularly effective because it uses a system-level approach to solving environmental problems. Applying our understanding of the relationships between abiotic and biotic components of an ecosystem can help us address grand challenges.
Unsolved Mysteries and Future Research:
- How can we optimize trophic transfer efficiency to waste less energy?
Blog-style Summary:
Ecosystem ecology is ecology on a very large scale with special consideration for many food webs and the concentrations of energy that are passed between trophic levels. Basically, ecosystem ecology observes the flow and transfer of energy between organisms and environmental surroundings through different forms and efficiency rates.
All natural processes must follow the laws of physics, and ecosystem ecology focuses on how we see nature doing exactly that. While we may have seen (and will continue to observe) bizarre and unique relationships between organisms and their environment, the ruler of this chaos still boils down to basic physics. Two ideas in physics regarding energy transfer are enthalpy and entropy. Enthalpy is the amount of energy that is invested into or released from a system during a reaction. Entropy refers to how unevenly energy is distributed in a system. When something that is disordered becomes ordered, it loses entropy, but the entropy of the entire system will not decrease. This is because work within the system has been performed to make something more ordered, and therefore the entropy has theoretically ‘moved’ but is still in the same system.
The first law of thermodynamics is that energy cannot be created or destroyed. This means that all existing energy is all there will ever be! But, it means that for humans to observe a change in energy, we are really just observing a change in the form of energy. In some cases, this is exothermic, which means energy or heat is being expelled or lost in a system. In other cases, it may be endothermic, meaning that the process absorbs heat during a reaction. Sometimes, when it seems like energy is coming from nowhere, it’s actually just potential energy turning into kinetic energy. Consider a bouncy ball being raised above the floor, about to be dropped. Without considering the physics involved, one could say that the ball has no energy because it’s really your hand that’s holding it up in the air. As soon as the ball is released though, it moves towards the ground and has kinetic energy– and you never even had to throw it towards the ground! That’s because the potential energy was almost ‘invisible’ because it’s just the force of gravity pulling the ball back towards the ground.
The second law of thermodynamics states that energy transfer is inefficient. Consider the ball again. The reason a bouncy ball eventually stops bouncing (if not touched) is because the energy that was potential energy can be transferred into kinetic energy and other forms, such as energy released when the ball hits the ground and makes a noise or comes into frictional contact with the ground surface.
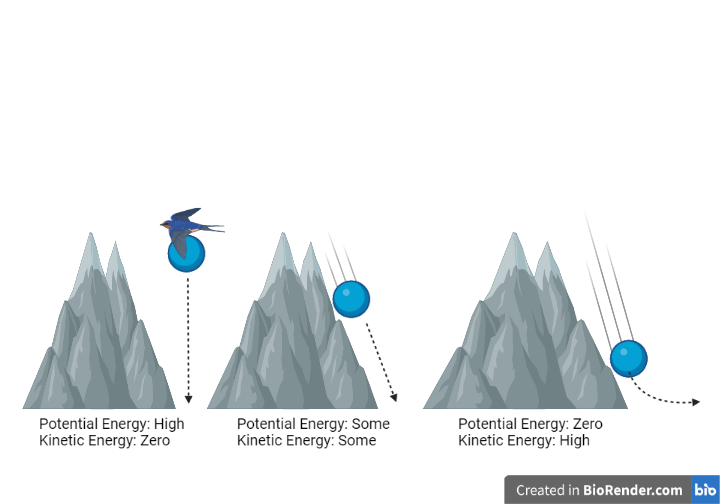
Within a food web, some of the most influential organisms are found within the lowest trophic levels. These organisms are primary producers, meaning they can produce their own nutrients through various means. Two examples of these unique types of organisms are classified as autotrophs and chemotrophs. Autotrophs are primary producers that produce organic compounds via carbon transfer. Chemotrophs are also primary producers, however, they obtain energy through the oxidation of electron-donating molecules. These two primary producing organisms produce nutrients through very complex systems that benefit their own systems and can impact global systems.
One way these unique primary producers are beneficial to global systems is through a concept known as Gross primary productivity or GPP. Gross primary productivity refers to the amount of carbon fixed by producers during photosynthesis. Essentially, when primary producers produce their own nutrients, they are fixing carbon dioxide from the atmosphere. These organisms utilize carbon dioxide to create nutrients and sugars that they need to survive. In turn, large numbers of primary producers, such as autotrophs and chemotrophs, have the ability to fixate or remove large amounts of carbon dioxide from the atmosphere. This proves to be a possible mitigation strategy when looking towards regulating anthropogenic carbon emissions.
Looking ahead at the implications of anthropogenic climate change, several studies have looked at how we can use the ecological pathway of carbon fixation to mitigate these implications. Currently, industrial carbon emissions have grown faster than natural systems have been able to keep up with them. This has led to us experiencing rapidly changing climatic conditions as a result of climate change. Biological systems and carbon pathways are simply unable to keep up with industrial burdens. However, many scientists have looked for ways to modify these natural systems to combat this challenge. For example, Gong et al.(2012) look toward the future of biotechnologies working alongside life sciences to improve upon carbon fixation pathways and produce gross primary productivity systems capable of keeping up with industrial carbon emissions.
Ecosystems, like all systems, are energetically inefficient. The conversion of light energy into organic matter has an energy tax, which is the respiration of autotrophs. Net primary production is the amount of organic matter that can be created by autotrophs. It is the usable energy that can be passed along the food chain. Thus, net primary production can be calculated by subtracting the respiration of autotrophs from the gross primary productivity. NPP is often expressed as units of biomass per unit of area and time.
For example, the net primary productivity of the Amazon rainforest would be measured by assessing the amount of carbon dioxide exchange that takes place over a given amount of time, which has been done using MODIS on NASA’s Terra satellite. The Terra satellite showed that the Amazon is actually the most productive during the dry season since the groundwater supply has been replenished during the rainy season and the skies are relatively clear of clouds, allowing more sunlight to penetrate the canopy (NASA).
However, GPP is not easily measured, especially at large scales. GPP actually cannot be precisely measured at all, and can only be assumed based on carbon exchanges in the ecosystem. There are carbon flux stations located across the globe that measure the exchange of CO₂ between the ecosystems and the atmosphere (Anav, et al.).
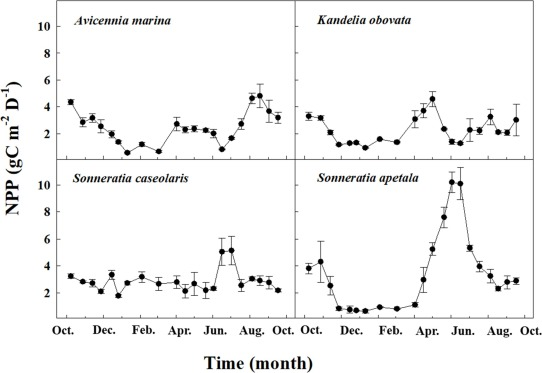
As stated in the earlier section, the transfer of energy from trophic levels is inefficient. Since energy transfer is inefficient, with every increase in trophic level, less energy is available. This is because organisms must use up energy to grow, reproduce, and carry on normal body functions necessary for life. By subtracting the energy lost in waste from the energy assimilated from food, you get assimilation efficiency. When you also account for respiration, this is the production efficiency. Secondary production is the amount of energy being used to grow new tissues and for reproduction. The amount of energy lost to secondary production, respiration (and transpiration, in plants), and waste is what makes systems energetically inefficient. So inefficient, in fact, that only ~10% of energy gets transferred from one trophic level to the next.
Spotlight on NC:
Ecosystem ecology pertains to the interaction between abiotic and biotic factors within an ecosystem. In order to understand how these interactions truly come into play it is important to understand the biotic systems that abiotic factors, such as sunlight and water availability, govern. The unifying factor between community ecology (focuses on species-level interactions) and ecosystem ecology is food webs. Food webs not only display the interactions between different species but also the energy level interactions.
Ecosystems transfer energy in multiple ways. The best way to understand energy in an ecosystem is to understand its origin. Primary producers absorb energy through photosynthesis or chemosynthesis and support higher levels of the food chain. Secondary production comes from consumers of primary producers, such as rabbits that eat grass. Primary and Secondary production in populations can be used to determine energy flow within ecosystems. Assimilation is the process of absorbing this energy while discounting the energy lost in waste. While energy is transferred to higher trophic levels, much of it is lost.
Understanding the laws of thermodynamics is essential for distinguishing the many roles and functions of organisms in an ecosystem. Potential energy is energy that is stored. An example of potential energy in ecosystems is ATP, which can power the other functions that also require energy such as respiration. Kinetic energy is energy in motion and is used to perform work. The First Law of Thermodynamics states that energy is neither created nor destroyed. From the ecological perspective, this can translate into the idea that energy must go somewhere. This concept can be modeled by the energy transformations along food chains and energy production systems. There are two types of reactions that may arise from this concept, only differing from how energy is transformed and or stored. For exothermic reactions, the reaction transforms energy from the system in the form of heat, light, or sound. An example of an exothermic reaction within an ecosystem maybe something like a forest fire, where energy is released in the form of heat. On the other hand, endothermic reactions absorb energy during the reaction. Photosynthesis is an example of an endothermic reaction because energy is transformed and stored in the form of ATP. Oftentimes, energy is measured thermodynamically as entropy and enthalpy. The best way to understand these terms and to branch into thermodynamics is to understand this figure:
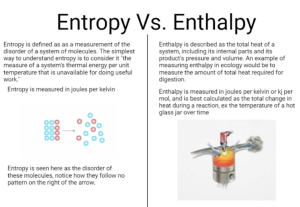
Along with these laws, ecosystem ecology takes into consideration trophic levels, energy transfer, food webs, biodiversity, individual species, and many other factors that work together in a functioning ecosystem. The first law of thermodynamics states that energy is not created or destroyed, however, this energy can be transferred. Transfers of energy take place in an ecosystem so that it can thrive and be self-sufficient. Primary producers (i.e. chemotrophs and autotrophs) are able to produce their own nutrients that are transferred up the food chain, as well as influence the climate around them. However, unlike autotrophs which produce organic compounds through carbon transfer, chemotrophs obtain their energy from the oxidation of electron-donating molecules. These primary producers are able to provide essential nutrients and benefits to other organisms in a food web.
Gross primary productivity (GPP) is a way to understand the benefits that these producers provide. Primary producers fix CO2 into the atmosphere when creating nutrients, and GPP is the amount of carbon fixed by primary producers during photosynthesis during a given period of time. Through this, these organisms are able to mitigate emissions and fixate or remove large amounts of CO2 from the atmosphere, an essential aspect of keeping our earth healthy. Any life process requires energy in order to be maintained. From photosynthesis in autotrophs, the light obtained is transformed into organic matter.
Net primary production (NPP) is the amount of organic matter that is created by these autotrophs that can be used for energy transfer through trophic levels. So, the calculation for the net primary production of autotrophs is the difference of how much carbon is fixed by primary producers (GPP) minus how much CO2 is released when metabolized (plant respiration).
There are many aspects that can limit NPP, including temperature, water, or light. So, NPP can vary based on the climate in which these organisms live. For instance, plant stomata must be open for the intake of CO2, so open stomata are what allow for transpiration to occur. Transpiration is dependent on water availability because plants can only transpire if they are able to replace the water lost. Thus, increased water availability warrants increased transpiration. Additionally, sunlight is essential for the process of photosynthesis and there is a proportional relationship between the amount of sunlight and energy available for photosynthesis. Therefore, the amount of these environmental aspects such as seasonal trends or growing seasons can affect the efficiency of NPP. For example, tropical areas near the equator have higher mean temperatures, rainfall, and canopy cover, hence having higher NPP. Cirino et al. (2014) examined the impacts of aerosols and deforestation on Amazon forests during each season compared to areas that were not impacted by anthropogenic effects. From this study, it was found that net carbon exchanges and primary productivity in these rainforests were significantly reduced because of biomass burning emissions. This is a good example indicating not only does the natural environment affect NPP, but humans as well.
Directionality in food webs tends to be dependent on where in the chain you are looking and which organisms are involved. Trophic interactions and energy movements that are unidirectional make up a majority of the forms of consumption and interactions in a food web. These food chains typically start at the autotrophic and primary producer level and move continuously up from there until the apex predator of an ecosystem has inherited a small amount of that original energy. What follows unidirectional food chains are the cyclical food chains, the component that recycles the energy back through the decomposing organisms from dead tissue. The cyclical component of a food chain can either be thought of as the decomposer sect of the food web, or the entire food web itself, as the movement of energy through the whole system is an open cycle, with some energy being lost as heat, while some energy is added from the sun.
The unidirectional and cyclical food chains are of particular importance in Eastern North Carolina, where there are many coastal plain and wetland ecosystems. Wetland ecosystems are among some of the most productive in the world (US EPA, 2015). Because of this, eastern North Carolina’s ecosystems provide a host of ecosystem services to both humans and to the greater environment westward. The reason for this is largely due not only to the size and complexity of the food webs in these wetlands but also the high efficiency of the energy movement around the food webs. Much of the detritus immediately has a large diversity of organisms ready to break nutrients down and the large concentration of vegetation makes the system highly productive.
How to Apply Ecology in a Changing World:
Wildfires have been a “hot” topic in modern times, particularly in places like California, Canada, and generally the American West, because of the size and scope of destruction they cause to humans and nature. The intensity, the size/spread of the fire, the amount of energy released, and the severity, or the damage, change, and ecosystem effects of fires, have increased compared to historical records of wildfire for a variety of reasons (Keeley 2009). A period of fire suppression and fuel overload started by campaigns of negative sentiment towards fire, an overall culture change due to post-Indigenous progression, and raised temperature and reduced moisture due to anthropogenic climate change have all contributed to the increase in fire effects (Roos et al. 2020 & EPA 2023). More recently, land managers have been looking positively at fire to reduce the intensity and severity of wildfires, preserve and improve native ecosystems, and promote Tribal ecoculture and traditional ecological knowledge (TEK).
People fear fire because of property damage and death, but fire-suppressed areas accumulate tremendous amounts of fuel loads that may cause much more damage than in areas with frequent fire regimes when a fire does break out. These fuel-heavy fires cause massive ecological damage such as soil instability and erosion, human and wildlife deaths, and increased CO2 release (Kelley 2009). On the other hand, if frequent burning was exercised, fuel loads would not accumulate to hazardous levels, and wildfires would be short-lived while still providing ecological benefits to the landscape. Fire is historically very common, healthy, and often necessary for North American ecosystems from the West to the East Coast. It opens up closed canopy forests promoting biodiversity and plant regeneration, provides increased high-quality forage and cover for wildlife, and facilitates ecosystem management and nutrient rotation (Keeley 2009).
Many species evolved to rely on fire to thrive in their habitats. Red Cockaded Woodpeckers (RCW), native to coastal NC and the Southeast, are endangered due to habitat loss and lack of frequent fire. They almost exclusively use mature longleaf pine savannas, which are heavily dependent on fire. RCW are ecosystem specialists, they build their nests by burrowing into live longleaf pines, and without many of these trees over a large area, they cannot persist (Kaiser et al. 2020). The survival and spread of longleaf pine ecosystems are dependent on frequent fire; when a fire is suppressed, more competitive species, like loblolly and shortleaf pine, move into the landscape and outgrow longleaf making it incredibly difficult for shade-intolerant longleaf pines to survive. The longleaf pines need wildfire for their cones to drop and germinate and have the ground cleared and soil exposed for optimal germination (Brockway et al. 1997).
Indigenous people have used burning in North America for thousands of years to steward landscapes for health and to provide for themselves. Land managers in California are beginning to understand fire’s place on the landscape and are implementing practices to improve health and contribute to the preservation of Tribal culture by managing for California black oak (Quercus kelloggii). These black oaks were historically used throughout the Sierra Nevada for making flour out of their acorns and were an important source of food for Indigenous peoples (Long et al. 2017).
Fire is also great at controlling invasive plant species and the overall vegetative structure. Controlled burns have been utilized for centuries for ecological restoration and land management. Fire helps to control invasive plant species by destroying their seeds and reducing the density of established vegetation, creating spaces for native plants to survive and thrive. Fire also plays a crucial role in nutrient cycling by promoting the release of essential minerals into the soil. This enhances soil fertility and stimulates the germination of certain plant species. Fire also prevents the accumulation of dead vegetation which reduces the risks of untamed wildfires and their damaging effects on ecosystems (Periara et al., 2021). When done under controlled conditions, prescribed burns are an environmentally friendly strategy for maintaining the health and balance of ecosystems and protecting biodiversity.

You can help with this by getting your Firefighter Type 2 certification and volunteering to help with prescribed fires in your state. You don’t even need a certification to burn on your land, but you should get fire safety training, obtain a burn permit from the local fire marshall, communicate with your neighbors, and develop a plan. You can also take it further and work for wildland fire crews with the BLM, USFS, or private organizations. These crews are more common in western states, and wildland fire work is hard, rewarding, and important work that we should promote as stewards of the land. Other than fighting fires and doing prescribed burns, crews also do fuel reduction by piling and removing debris/fuels in forests and woodlands to prevent intensity/severity from getting out of control as a result of naturally started i.e. lightning, fires. They also do fireline preparation by clearing corridors in forests so that fires are less likely to spread uncontrollably. Some of these firelines are used as recreation trails, and some trails are used as firelines, throughout the year.

Fire is important and not all bad. We need to continue to spread the word and integrate fire regimes in our land management to conserve our native ecosystems. The more we learn about Traditional Ecological Knowledge and fire’s role in the evolutionary history of our country, the healthier we can make our ecosystems. Prescribed fire is beneficial, we need it more widespread and more often to prevent catastrophic destructive fires. Some ecologists have begun using social media to spread the word, like the Native Habitat Project and others, in hopes of raising more awareness among the public.
References:
Brockway, D. G., & Lewis, C. E. (1997). Long-term effects of dormant-season prescribed fire on plant community diversity, structure and productivity in a longleaf pine wiregrass ecosystem. Forest Ecology and Management, 96(1), 167-183. https://10.1016/S0378-1127(96)03939-4
EPA (2023) Climate Change Indicators: Wildfires. EPA.gov. https://www.epa.gov/climate-indicators/climate-change-indicators-wildfires
Kaiser, A. L., Soulé, P., van de Gevel, S. L., Knapp, P., Bhuta, A., Walters, J., & Montpellier, E. (2020). Dendroecological investigation of red-cockaded woodpecker cavity tree selection in endangered longleaf pine forests. Forest Ecology and Management, 473, 118291. https://10.1016/j.foreco.2020.118291
Keeley, Jon E. (2009) Fire intensity, fire severity, and burn severity: a brief review and suggested usage. International Journal of Wildland Fire 18, 116-126.
https://doi.org/10.1071/WF07049
Long, Jonathan; Ron W. Goode, Raymond J. Guitteriez, Jessica J. Lackey, M. Kat Anderson. (2017) Managing California black oak for Tribal ecocultural restoration. Journal of Forestry. 115(5): 426-434 https://www.fs.usda.gov/research/treesearch/53599#
Paulo Pereira, Igor Bogunovic, Wenwu Zhao, Damia Barcelo, Short-term effect of wildfires and prescribed fires on ecosystem services, Current Opinion in Environmental Science & Health, Volume 22, 2021, 100266, ISSN 2468-5844, https://doi.org/10.1016/j.coesh.2021.100266.
“Prescribed Fire – Oklahoma State University.” Prescribed Fire | Oklahoma State University, 19 Dec. 2019, extension.okstate.edu/topics/environment-and-natural-resources/rangeland-management/prescribed-fire/.
Roos, Christopher I., Tammy M. Rittenour, Thomas W. Swetnam, Rachel A. Loehman, Kacy L. Hollenback, Matthew J. Liebmann, and Dana Drake Rosenstein. (2020). “Fire Suppression Impacts on Fuels and Fire Intensity in the Western U.S.: Insights from Archaeological Luminescence Dating in Northern New Mexico” Fire 3, no. 3: 32. https://doi.org/10.3390/fire3030032
Featured Ecologists:
Silvia Alvarez-Clare, MS, PhD
Dr. Alvarez-Clare dedicates her research to understanding how environmental changes affect plant communities, with a particular emphasis on trees. Through extensive monitoring, experimental studies, and ecological methodologies, she investigates the impacts of shifting climate patterns, human land use, and soil nutrient dynamics on forest ecosystems. A cornerstone of her work is the EFFEX program, which she helped establish in 2007. This long-term study examines how increased nitrogen and phosphorus availability affects various forest processes. By adding these nutrients to designated 30 x 30m plots, researchers observe changes in forest productivity, organic matter breakdown, and soil greenhouse gas emissions. At The Morton Arboretum, Dr. Alvarez-Clare leads critical conservation initiatives to protect endangered tree species. Her approach emphasizes practical applications, transforming research findings into actionable policies and management strategies. As the leader of the Global Conservation Consortium for Oaks, she works tirelessly to prevent oak species extinction worldwide. One of her innovative strategies is the development of a “metacollection” – a scientifically coordinated network of living oak collections distributed across multiple locations. This approach addresses the practical impossibility of preserving all oak species at a single site while ensuring their survival through collaborative conservation efforts that engage stakeholders around the world.
Carla Atkinson, PhD
Dr. Carla Atkins studies the ecology and processes of aquatic ecosystems, with a focus on rivers and wetlands in the southeastern US and along the Gulf Coast. She also investigates the interactions between aquatic and terrestrial ecosystems in the context of feedback within those aquatic systems. A major focus of her research is the impacts that community structure and individual species can have on ecosystem function. For example, in the study featured in the above Biorender, the effects of the presence of freshwater mussels in stream ecosystems on nutrient cycling was investigated using a field observational study. Freshwater mussels are highly endangered and it was found that they are necessary for regulation of nutrient cycling. Other research has focused on the role of amphibians in linking terrestrial and aquatic ecosystems, the effects of stressors on mussels, and microbiomes in streams. She has found that biodiverse communities are linked to ecosystem function. This research is performed in order to both enhance scientific knowledge and to promote the conservation of biodiversity on an ecosystem level. As anthropogenic change increasingly harms important freshwater ecosystems, it will be important to have a dynamic understanding of potential effective conservation in respect to organisms that maintain ecosystem processes.
Karl Castillo, PhD
Dr. Karl Castillo’s research focuses on temperate and tropical corals and their various stressors. He seeks to understand how the physical environment surrounding these corals impacts their performance. One of the most pressing issues that Dr. Castillo’s lab investigates is global change. Rising temperatures and ocean acidification pose serious threats to coral reefs worldwide, but more research is needed to fully understand these threats. Dr. Castillo also investigates the past and future of coral reef systems. He studies how corals have been affected by stressors in the past and uses those data to determine how the future could look for these important systems. Dr. Castillo conducts research off the coasts of North Carolina, Florida, and Belize using a variety of different research methods. Recently, Dr. Castillo has published papers explaining how different corals are affected by disease and how coral skeleton density has changed over time. This research is particularly important because coral reefs provide habitat for many different species, making them biodiversity hotspots. Their conservation is very important for marine biodiversity as a whole. Dr. Castillo is also committed to education, serving as an associate professor at the University of North Carolina, Chapel Hill.
Sharon Hall
Sharon Hall is an ecosystem scientist at Arizona State University. Her early work considered nitrogen emissions and how they affected the surrounding environment. She focused primarily on terrestrial ecosystems, especially tropical forests. Her research found that ecosystems react differently to having an overabundance of nitrogen, but that it often leads to ecosystem decline in the long-term. Her current research focuses primarily on the interactions between humans and the environment. She looks at how humans and urbanization affect the environment. Some of the things she studies include the biodiversity of cities, how air pollution affects the health of an ecosystem, and how people treat wildlife in their environment. She looks at how all of these factors affect the functioning of ecosystems. She also studies how areas that are still developing react to the rapid environmental change that comes with urbanization. Her research is done in the context of informing land management strategies in urban or residential settings.
Katie Martin
Dr. Martin leads the Watershed Ecology lab group at NC State, and her research is primarily concerned with ecosystem services provided by forests. She is especially interested in the interactions between forests and water (ecohydrology), and also dabbles in (wild)fire ecology and its impacts on forested ecosystems. As a member of the Center for Geospatial Analytics at NC State, Dr. Martin does a lot of work with GIS software and modeling to understand these ecosystems. This allows her to study ecosystems across the Southeastern United States (and the entire world if desired), since she is not limited in scope to sites she can physically sample (although she has worked at Coweeta Hydrologic Laboratory in the mountains of NC). Some of her recent work has focused on the impacts of climate change on forest water resources (specifically the effects of longer growing seasons – causing increased transpiration and water stress in plants) and the effect that forests have on downstream water quality (shown in the Biorender above – forested watersheds have the highest water quality). Her work sheds light on how we can manage these systems for water resources and biodiversity, and it has implications for sustainable development. Dr. Martin also maintains an interest in promoting environmental justice and increasing diversity in forestry.
Nalini Nadkarni, PhD
Dr. Nadkarni studies forest canopy communities with a specific interest in how epiphytes create a nutrient-rich mat beneath their roots which provides the trees with resources despite the nutrient-poor soil on the forest floor. She is well known for using mountain climbing equipment to reach the Costa Rican tropical forest canopies and taking inventory of the species there. Her most well-known work comes from her studies of tropical montane forests in Costa Rica and the diversity of epiphyte life. Her methods include sampling epiphytes through the elevation gradient and determining how elevation, land-cover type, and microclimates influence the taxon richness and biomass. She found that epiphyte community composition differed between elevation (with higher taxonomic richness at higher elevation)and land-cover type (with differences in family and functional-group biomass). In another study on epiphyte drought avoidance in montane cloud forests, Dr. Nadkarni found that canopy plants can mitigate water loss through internal storage or the use of cloud water. Dr. Nadkarni has another interest in habitat fragmentation and how that affects epiphyte biodiversity. She argues that climate change will result in clouds forming at higher elevations and epiphyte communities will shift towards a more drought-resistant species composition. Her research relates to our discussions on metapopulations & landscape ecology as well as patterns of biodiversity & species interactions.
Pamela Matson, PhD
Pamela Matson is heavily involved in the sustainability side of ecology, with a bulk of her research revolving around anthropogenic impacts on the environment. Much of her research revolves around how deforestation and pollution directly impact the atmosphere and climate, along with the impacts these anthropogenic changes have on the carbon and nitrogen cycles. Pamela Matson is married to Peter Vitousek, who is also an ecologist that focuses on the nitrogen cycle. Much of Matson’s research is conducted in the style of a case study, looking at one specific area, finding a problem in the sustainability of the environment there, and trying to locate the source of the issue, or a possible solution to the negative impacts the local environment is facing. A good chunk of her work is based on Yaqui Valley in Mexico, the birthplace of the Green Revolution, where she has conducted numerous studies on how to optimize and sustainably practice agriculture without harming the soil and making the land unusable for future generations. Other impactful research she has conducted was a study on the deforestation and pollution happening in the Amazon, and how these changes were directly impacting the atmosphere. A majority of her work relates to our unit of landscape ecology, since she is looking at a specific area and searching for possible ways to ensure the survival of the local ecosystems while also attempting to find a use for the land that is beneficial for humans and is sustainable. Pamela Matson has written two books, titled “Pursuing Sustainability: A Guide to the Science and Practice,” and “Principles of Terrestrial Ecosystem Ecology”.
Amina I. Pollard
Amina Pollard studies nutrients and toxins in all kinds of aquatic ecosystems to diagnose their health and human influence. Her goal is to determine the quality of US lakes. Dr. Pollard and her team have found that ecosystems with increasing toxins and eutrophication are less productive. She also studies anthropogenic influence on water systems effects can affect the entire regional climate and the physical features of the ecosystem. Using this research, we can predict how much human involvement is sustainable in an aquatic system and hopefully apply in nationwide. She complies mass data to make these predictions.
Scott Roberts
Scott’s first publication studied the effects of hemlock (wooly adelgid) mortality in Great Smoky Mountain National Park on water quality and riparian ecosystems in first and second order streams. Between six pairs of hemlock and hardwood streams with similar spatial characteristics accounting for watershed size, flow rate, terrain, geology, forest structure, etc., they found that forest canopy type had no significant influence on most stream conditions (temperature, nitrate concentration, pH, discharge, and summer photosynthetically active radiation and foliar cover) suggesting that the loss of hemlock trees in the Smoky Mountains would not have a significant effect on hydrology within the region. The only significant difference was noted where hardwood understories lacked dense rhododendron cover there was nearly 10x more light penetration compared to hemlock stands with no dense understory. Their discussion suggests that a threat to hardwood succession in fading hemlock-dominated stands is aggressive colonization of rhododendron, which may crowd out hardwood saplings. This possibility warrants study into how the hydrologic characteristics of rhododendron-dominated watersheds differ from those that are historically hemlock-dominated. These differences have implications for not only abiotic characteristics, but also for specialized, biotic communities in the hemlock forests of GSMNP, like trout and endemic amphibian species.
I think Scott’s current work at a local non-profit organization is very important to advocacy for science and conservation. Mountain Studies Institute has grown some over his time there and conducts research and public outreach throughout their Southwest Colorado region. They provide public education and community science opportunities, which I have taken part in, and partner with other organizations, state and federal agencies, and education institutions to complete or contribute to important projects. Projects that Scott is involved in cover river health, water quality, and benthic macroinvertebrate health in streams in the San Juan Mountains, especially those in proximity to mine reclamations and heavy recreation activity. MSI conducts annual E. coli testing at various elevations in alpine streams to monitor how local tourism and livestock grazing affects aquatic health and associated communities. Scott, MSI, and community partners survey effects of wildfire, climate change, and other temporal and spatial conditions on the Animas and Colorado Rivers as well as Hermosa Creek at a more local level. They have conducted and published benthic macroinvertebrate surveys following severe wildfires in Hermosa Creek as well as monitoring post-fire vegetative succession across several years.
Brad W. Taylor
Brad Taylor’s is a freshwater ecologist and the research his lab “uses rivers as a study system to discover the degree to which a few individual species affect the 1) phenotypic traits of other species, 2) community interactions, and 3) ecosystem functions, such as carbon flow and nutrient cycling. He is also interested in interdisciplinary research blending ecological and economic concepts to further our understanding of the proliferation and control of invasive species.” Just this week in class we talked about community ecology and that is one of the ecological concepts that he explores he also looks at life histories. One of his major findings come from a collaborative research work called Consequences of Climate-Induced Range Shifts on Multiple Ecosystem Functions where the conclusion of the research states “In the broader context of range shifts, invasive species, or other compositional shifts that modulate ecosystem functioning, our results demonstrate that dominant and subdominant resident species can regulate ecosystem processes throughout sequential range expansions by species with similar life histories. In contrast, range expanding species with differing life histories can change relative contributions of species to ecosystem processes”. I also think it is really cool how he is a professor here at NC State.
Student Contributors:
Note Outline: Ilene R Doyle, Yinan Ding, Elizabeth Sauser, Sasha Pereira
Blog Style Summary: Eileen Tan, Madison Shrader, Amelia Barrow, Katie French
Spotlight on NC: Helena Jolly, Dawn Trivette, Alex Tung, Garrett Morgan
How to Apply Ecology in a Changing World: Alex Yates, Madison Burnette, Sarah Alanbagi
Featured Ecologists: Jordan Holcomb, Dorian Hayes, Ben Regester, Madigan Clossick, Teddy Sarian
amount of internal energy in a compound
A measure of disorder, or how evenly energy is distributed in a system
a reaction that releases heat into the surroundings (the system loses energy)
a reaction that absorbs energy/heat into the system
stored energy
energy in motion, performing work at expense of potential energy
organisms that produce organic compounds via carbon transformation (e.g., carbon dioxide to sugar)
organisms that obtain energy through the oxidation of electron donating molecules in the environment
the total amount of carbon fixed by producers during photosynthesis
amount of organic matter created from photosynthesis, expressed in units of organic matter per unit area per unit time
NPP = (Gross primary productivity) - (Respiration by autotrophs)
moving nutrients into cells where they are used to perform bodily functions
growth of new tissues and reproduction
water that is lost from leaves into the surrounding air