Chapter 5: Patterns of Biodiversity and Species Interactions
Vocabulary:
- Alpha diversity: a metric to quantify the diversity of types of organisms and/or their relative abundance
- Beta diversity: variation between communities, resulting either from differences in membership (which species are present / absent) or in the relative abundance of species
- Biodiversity: variety and variability of life on Earth
- How much variation there is within and between different types of life
- The Open Tree of Life estimated 2,391,916 species on Earth as of fall 2015
- This estimate was up to 2,384,572 species in fall 2024
- How much variation there is within and between different types of life
- Chao richness estimator: diversity index for estimating the total number of species, based on the observed species in a given sample. Developed by Anne Chao in 1984.
- Chao1 incorporates the number of species and their relative abundance
- Chao2 is incidence-based, meaning it only takes presence/absence into account
- Diversity dips: areas where biodiversity decreases, particularly in desert areas
- Equatorial peak: biodiversity tends to be highest at/around the equator; this pattern is true for both terrestrial and aquatic species
- Mass extinction event: An event in which 75% or more of all species go extinct within a period of 2 million years
- Rarefaction curve: a method of plotting the total number of different species have been encountered in a given number of samples, that helps ecologists estimate the species richness in a sample or community of interest, and to gauge how much sampling is “enough” to get an accurate picture of the community
- Relative abundance: the proportion of a community that is represented by a single species
- relative abundance = (total number of individuals belonging to one species) / (total number of individuals in the entire community)
- Richness: a measure of alpha diversity that quantifies the number of species in a sample or community of interest
Outline of Notes:
How do we measure diversity?
Estimating Richness:
Species richness can be estimated by calculating diversity indices:
- Rarefaction curve: a graphical representation of how many new species are observed per sampling effort
- First exponential: new sequences every time
- Second lag: resampling dominant species stick out more but some rarer species are being still discovered
- Richness plateaus when ‘return on investment’ decreases
- Example from class: Erin created an Excel spreadsheet that graphs a rarefaction curve for individual letters sampled from the class syllabus.
-
Figure 1. Rarefaction curve documenting the number of “species” (different letters) sampled from a “community of interest” (the Applied Ecology syllabus)
-
- Fossil record: the number of species present in the fossil record in a given area can give an estimate of the species richness
- DNA sequencing: DNA can be sequenced at different geological depths in order to determine the occurrence of particular genes in an environment
- Chao1: diversity index for estimating number of species for total population based on a given sample
Past Extinctions:
It is predicted that 99% of all species that have ever existed are now extinct. Since life began on Earth, there have been 5 major mass extinction events:
- End-Ordovician: ~443 million years ago
-
- 86% of species and 57% of genera went extinct (e.g. many corals, brachiopods, and trilobites)
- End-Devonian: ~359-380 million years ago
-
- 75% of species and 35% of genera went extinct (e.g. most marine invertebrates)
- End-Permian: 251 million years ago
-
- 96% of species and 56% of genera went extinct (e.g. almost all marine species)
- End-Triassic: 201 million years ago
-
- 80% of species and 47% of genera went extinct (e.g. many terrestrial vertebrates)
- End-Cretaceous: 65.5 million years ago
-
- 76% of species and 40% of genera went extinct (e.g. dinosaurs and ammonites)
→ Biodiversity experiences exponential growth after mass extinction events, indicating niche space becoming available

- Holocene: began ~12,000 years ago, after the end of the last ice age
- Anthropocene: 1950- present; began with dramatic and unprecedented increase in human activity
-
- Humans have caused 75% of extinctions since the 1600s
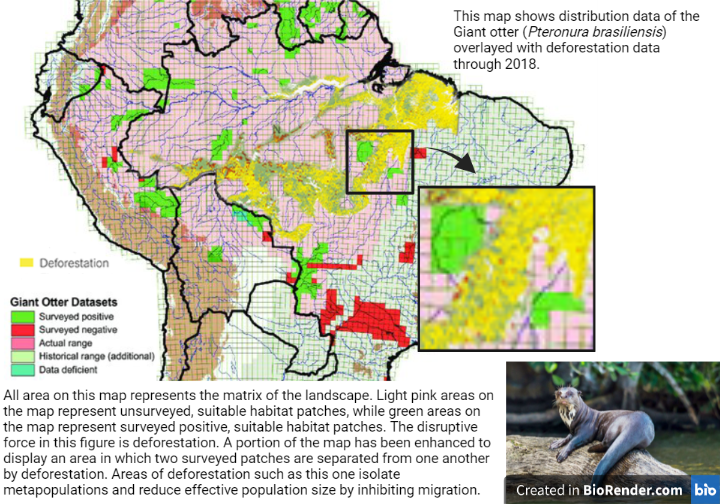
Drivers of Biodiversity:
- Age of Community
- Climate Stability
- Energy Availability: evapotranspiration can be used as a proxy for photosynthesis at the base of the food chain
- Increasing plant energy available = increasing animal diversity
- Spatial Heterogeneity
- Habitat heterogeneity increases the number of species (alpha diversity) and variation between communities (beta diversity)
- Ecosystem Productivity
- Net primary productivity by plants increases overall ecosystem productivity
- When environmental conditions are favorable for photosynthesis, many tree species can be supported which leads to greater plant diversity over time and creates niche space for greater animal diversity
- Land Area
- Migration: can cause diversity to vary seasonally
Biodiversity Patterns:
- Patterns in North America:
- Endemic species are concentrated in the Southeast
- Mammals have more biodiversity in the West and North than the Southeast
- Most of the protected lands in the US exist in the North and West
- Conservation and land protection in the US didn’t become popular (in colonial cultures) until relatively recently, and the southeast is rich with farmland that is used for agriculture instead of protection for wildlife.
- Diversity is highest near water sources, specifically around the Mississippi River
- Global Patterns:
- Biodiversity increases near the equator
- Amphibian diversity is highest in South America and lowest in desert areas
- Bird diversity is highest in tropical areas
- Marine environments have high numbers of threatened species worldwide, and Brazil and the US have high numbers of threatened bird species
- Marine biodiversity is greatest in the Pacific, near Southeast Asia and Australia
- Species Diversity Distributions
- Terrestrial diversity increases as you near the equator (latitudinal gradient) and decreases in deserts
- “Equatorial peaks” and “Desert drops”
- Most species follow this pattern
- Exceptions: species whose diversity follows the opposite or whose diversity is more closely correlated with longitudinal gradients
- Terrestrial diversity increases as you near the equator (latitudinal gradient) and decreases in deserts
Key Takeaways
Overarching Themes and Unifying Concepts:
- Species richness and diversity can be estimated using refraction, DNA sequencing, and by looking at the fossil record.
- There have been 5 mass extinction events since life began on Earth: End-Ordovician, End-Devonian, End-Permian, End-Triassic, and End-Cretaceous.
- We are in the middle of the 6th mass extinction, the Anthropocene extinction.
- Environmental factors and extinction periods have shaped the species diversity and species richness present on Earth today.
- Drivers of biodiversity in an area include age, climate, energy availability, habitat heterogeneity, ecosystem productivity, land area, and migration.
- Biodiversity generally increases as you go towards the equator and decreases as you approach the poles and in deserts.
How might this information be applied to address grand challenges?
- Considering diversity indices and locating biodiversity hotspots can help us to determine where to allocate resources for conservation
Blog Style Summary:
When someone asks you “What does biodiversity mean to you?” many definitions may come to mind. Probably the simplest definition of biodiversity is: the variety and variability of life on Earth. Now, just how much biodiversity is there on our planet? In 2008 there were 1.6 million known species, in 2009 there were 1.9 million, and in 2015 there were 2,391,916. As of fall 2021, there were 2,392,042 known species. However, there are estimated to be 8.7 million eukaryotic species and up to 1.6 million prokaryotic species in total. This means that a majority of life on Earth has yet to be found or classified!
The Earth hasn’t always been like this, however. There have been 5 mass extinction events, or events in which over 75% of species have gone extinct within a 2 million year period since life began on this planet (see Figure 2). However, mass extinctions don’t spell total doom for biodiversity. Exponential growth in species has occurred before some, and after all, mass extinction events. This illustrates that while mass extinction events do imply doom for many, they also open up niches for other species to thrive and evolve. For example, we wouldn’t have those cute and fluffy mammals we all love if dinosaurs hadn’t gone extinct!
You may be wondering how the amount of species we have on Earth is determined in the first place. Biodiversity can be estimated through multiple methods: rarefaction, fossil records, and DNA sequencing. Chao1 is a method used to estimate the richness of species within a population based on a sample from the community. Rarefaction is another method used to assess species richness from the results of sampling. Figure 4 below shows a rarefaction curve. Section A of the curve shows that new species are being found with every sample taken, section B shows that there are some repeat species found with each sample but there are still new ones being found, and section C shows that you are only finding repeat species so all of them have most likely been found. Rarefaction curves can be used to gauge how much sampling is “enough” to get an accurate picture of the species composition of the community.
Figure 4. Rarefaction curves are used to plot the discovery rate (# new species identified) versus sampling effort (total # samples collected). Early sampling efforts are characterized by rapid discovery rates, with new species detected in almost every sample. As the more abundant species are resampled in subsequent efforts, discovery lags. Eventually, new species are detected only occasionally (when a rare species is sampled), resulting in a plateau in the rarefaction curve.
Ever wonder why a red maple tree isn’t found in the desert or why moose don’t live in North Carolina? It is because environmental patterns such as water availability, temperature, climate, geology, habitat connectedness, and the age of a community, are highly variable across geographic regions. A desert is too arid for a red maple tree and North Carolina is too hot for moose. These differences in environmental conditions lead to higher global biodiversity because no one species can be perfectly adapted for this wide range of conditions. Other conditions, such as energy availability and ecosystem productivity, also influence species diversity. Land areas with conditions ideal for plant photosynthesis- ample sunlight, frequent rainfall, and moderate temperatures- support plant growth. In turn, high plant growth and high plant diversity are able to support more diverse fauna. The same can be said about marine environments. Shallow water areas receive more sunlight and nutrients from upwellings that in turn support more plant growth. More plant growth supports a higher diversity of fish (Figure 2).
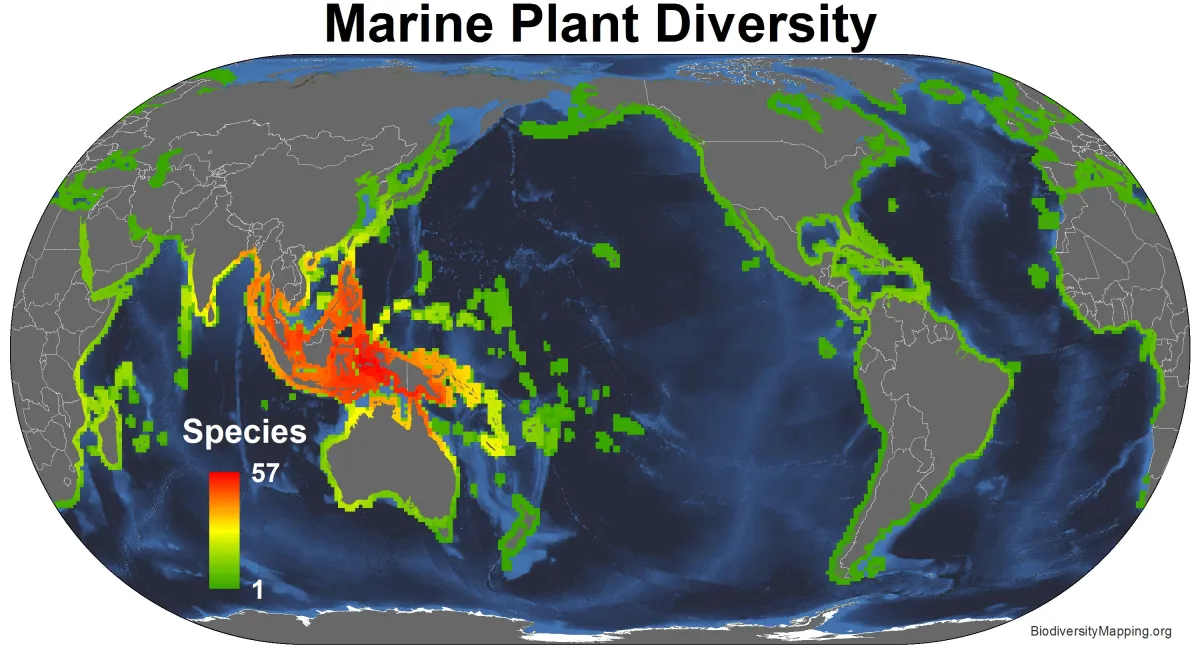
When looking at various maps of diversity across the globe, we notice some similar trends and patterns. One noticeable trend is that diversity is often highest towards the equator (sometimes called an equatorial peak). This is likely due to a larger input of energy (sunlight) allowing for greater plant productivity, which benefits higher trophic levels.
Rainforests provide a dramatic example of biodiversity in tropical and subtropical areas. On the opposite end of the spectrum, biodiversity decreases in desert regions; this is known as a diversity dip. The harsh climate and geography of desert regions do not allow many species to survive.
Understanding species diversity patterns will be important as climate change alters environmental conditions and shifts habitat ranges. Changes in biodiversity can be a symptom of change in ecology, health, climate, or other biotic and abiotic factors. Climate change, habitat loss, and other anthropogenic factors have all contributed to a declining global biodiversity. Understanding how and why species exist where they do, and how environmental changes are impacting them, will help us better conserve the remaining biodiversity we have left.
Spotlight on NC:
Green Salamander: A Lesson in Biodiversity
One fact that always amazes me is that 99% of life that has lived on this planet has gone extinct. As big and vast as all the current life on Earth seems, it is only a tiny fraction of all the different kinds of life that have ever existed. The variety of life that currently exists on Earth is referred to as biodiversity. This word is probably giving you déjà vu as it is constantly being thrown around in the news, specifically in stories about how humans are causing global declines in biodiversity. As you can probably guess, declining biodiversity is a very bad thing. Declines in biodiversity mean ecosystems are less productive, less healthy, and more unstable. This leads to cascading effects across trophic levels and on the global scale.
While we are usually guilty of only thinking about biodiversity in terms of our favorite zoo animals, there are almost 2.4 million species of all kinds of life out there. While this may seem intimidating, much of this number are microscopic forms of life, which are still very important to all other walks of life. In this blog post, we’ll be taking a deep dive into a tiny little species in North Carolina that shows the ginormous concept of biodiversity and why it is so important.
Deep in the Appalachian mountains, at the junction between North Carolina, Georgia, and South Carolina, you may be lucky enough to find the Green salamander (Aneides aeneus) [Fig 1.]. They belong to the Plethodontidae family of lungless salamanders. The Green salamander is somewhat unique among other Aneides species however, as it and only one other species, the Hickory Nut Gorge green salamander, are found along the Eastern portion of the United States [1]. This species prefers to spend the majority of its time inside moist rocky crevices within the mountains, which provide them with protection while keeping them moist so they can breathe through their skin [2]. A 2005 study found that arboreal or woody spaces, namely those with hardwood trees, also provide important habitat for these salamanders. While their damp, rocky outcrops keep them safe during the colder winter months, when spring comes the salamanders migrate towards nearby trees and the surrounding leaf litter and remain primarily arboreal throughout summer [3].
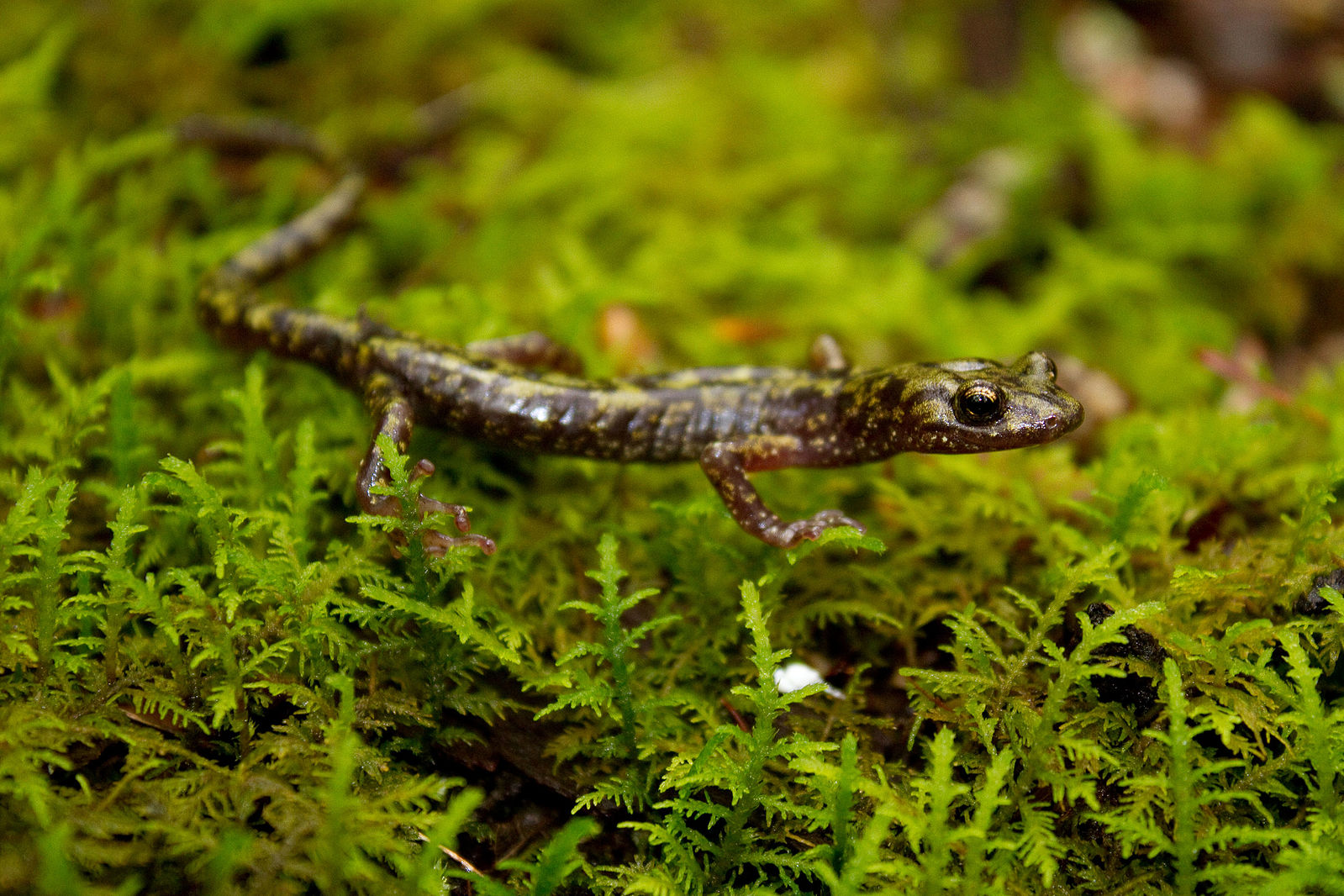
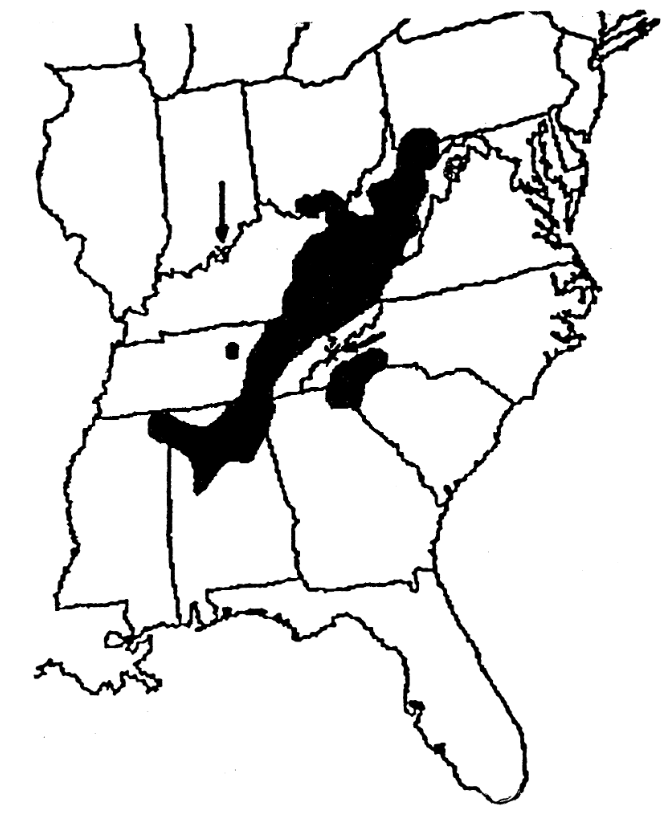
Ranging from about 8-12 cm long, the Green salamander is a medium-sized member of its family, with square-tipped toes that aid them in climbing. This enhanced ability to climb allows them to be excellent insectivores, feeding on spiders, ants, mites, and other arthropods [4]. Despite their excellent hunting abilities, the Green salamander still faces predation risks of its own- mostly from snakes and spiders, which are able to fit into the same rock crevices the salamanders call home. Predatory attacks such as these aren’t exclusive to adult salamanders- they threaten their eggs as well. Unlike most other salamander species, Green salamanders undergo direct development in the egg, hatching as a “mini” adult. This differs from most other amphibians as their eggs do not need to be laid in a water source, and their young skip the typical aquatic larval stage that most salamander species experience [5]. This strategy for reproduction only further highlights the need for these damp rock crevices, as their role in keeping the eggs moist is crucial for offspring survival.
Green salamanders in particular have faced a lot of challenges over the last few decades. Population estimates from across their ranges have shown a decline of up to 98% in some areas, compared to their 1970 populations [6]. The causes of their decline are likely a combination of habitat fragmentation, overcollection by scientists, climate change, and, to a degree, outbreaks of chytrid fungus – a deadly fungus that has been decimating amphibian populations globally. Climate change, namely adverse weather conditions here, seems to be a major cause of the decline of this species. Temperature-related mortality was proposed as the likeliest cause for the die-offs of these salamanders, and the overlap between the winter of 1996-1997 and a large proportion of salamander deaths points to this. All of these working together against the Green salamander have led to an IUCN listing as “near threatened”, along with varying local classifications, such as Indiana’s “state endangered” listing [7].
Hickory Nut Gorge Salamander
Deep within the Smoky Mountains lies 12 million years worth of evolution and one impressive biodiversity hotspot in North Carolina [8]. Hickory Nut Gorge is what some have phrased, “an ecological treasure” and is home to 37 rare plant species and 14 rare animal species [9]. Ecosystem productivity is particularly high in this area of sheer granite cliffs and undisturbed forest. The diversity of salamanders in North Carolina is expansive, with 64 species found statewide, however, Hickory Gorge hides a small, green emerald that has isolated itself within the rocky crags [8].
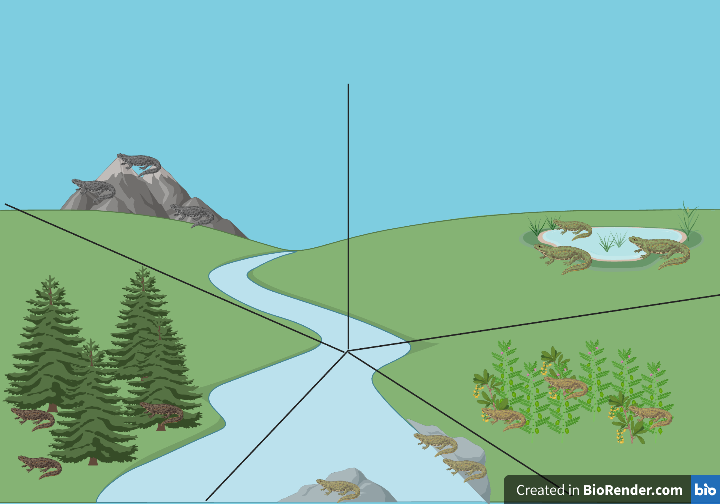
After fighting off the sheer panic of 14 miles worth of steep cliffs and tumultuous water, one may be lucky enough to spot the Hickory Nut Gorge green salamander (Aneides caryaensis) [Fig. 3]. Discovered in 2019 by an eager scientist named J.J. Apodaca, its bright green spots, adhesive toe pads, and long arms make it suited for the rugged terrain [10]. The Hickory Nut Gorge green salamander (phew quite a long name for such a little guy!) can breathe without lungs and is endemic to the gorge [8]. While its name may insinuate that it’s just another green salamander, due to environmental heterogeneity it is actually a genetically distinct species [8]. Unfortunately, its small ecosystem range and specialization that gave it its name makes it particularly vulnerable to climate change, anthropogenic effects, disturbances, and inbreeding, leading it to have an IUCN classification of “critically endangered”[11, IUCN]. Petitions have been formed in an effort to provide federal protections for the Hickory Nut Gorge green salamander under the Endangered Species Act. Hopefully, this will help mitigate its rapid decline and prevent its extinction [11].

You may be wondering why it’s so important to conserve this small, endangered species, after all, it lives in one of the most isolated parts of the Smoky Mountains. Well, the Hickory Nut Gorge green salamander plays a key role in ecosystem biodiversity and food web interactions [11]. It assists in nutrient cycling, reduces pests, and links invertebrates and vertebrates together [11 & 10]. Without this salamander, interspecific interactions may decline and the biodiversity of the Smoky Mountains may suffer. Though the Hickory Nut Gorge green salamander is only one species in a region of expansive biodiversity, it is a crucial key in protecting the livelihood of its natural habitat.
How to Apply Ecology in a Changing World
Batrachochytrium dendrobatidis, also known as chytrid fungus, is a parasitic fungus affecting frogs worldwide. It was first analyzed in the late 1900s, though it is suspected that this disease originated in Africa in the 1930s and spread through trade of the African clawed frog [1]. Chytrid fungus infection in amphibians can lead to Chytridiomycosis, an often deadly disease. The fungus destroys the keratin layer in frog’s skin as well as the nervous system. This makes it hard for frogs to breathe as they respire through their skin. It may also cause abnormal behavior such as sluggishness, reduced appetite, staying in the open, and holding the legs away from the body instead of tucked in closer [2]. The chytrid fungus is found in both soil and water but can also be found on plants or insects [3]. It reproduces asexually and disperses through water [3]. The disease spreads to frogs from contact with contaminated waters or from human activity such as improper handling of frogs and equipment when working in affected areas. It is recommended that all clothes, shoes and equipment be disinfected thoroughly [3]. It is not guaranteed that all frogs at affected sites will become infected, but certain life histories can put species at risk. For example, frogs that breed in year round water sources (as opposed to ephemeral pools) are more likely to come into contact with chytrid fungus as it is probable that the water is already contaminated [3]. Additionally, species with lower fecundity are at greater risk as their populations cannot recover as quickly compared to species that produce more offspring [3].

Amphibian biodiversity shows an equatorial peak, with the greatest numbers of amphibian species found in warm, moist environments like the Amazon rainforest [4]. Year-round tropical conditions are great for frogs, but they also provide an ideal environment for chytrid fungus, which has devastated tropical frog species. Frog populations in temperate environments are often affected differently than tropical populations due to the more variable and seasonal environmental conditions [5]. Temperature is possibly a factor in chytrid fungus occurrence, but studies have provided inconsistent results so far [6]. Because of the variability in habitat conditions across different amphibian populations, there is no single solution that will save every population from chytrid fungus. Mitigation strategies must be tailored to populations based on the characteristics of the species and habitat.
It is estimated that chytrid fungus may have played a role in the decline of over 500 amphibian species, and resulted in the extinction of 90 of those species. Severe declines in amphibian biodiversity can cause many negative effects across ecosystems, due to amphibians’ involvement in aquatic and terrestrial food chains as both predators and prey. Some studies have shown that snake species richness decreases when chytridiomycosis causes large die-offs of amphibians, which are important prey for snakes [4]. Given the trophic cascades that can be caused by amphibian extinction, finding ways to reduce chytrid-related mortality is of utmost importance.
Although there are ongoing efforts to treat chytrid fungus, there are also ecological solutions that can decrease the spread and virulence for amphibians. These solutions focus on habitat management control, toward the conservation of whichever amphibian species lives in the area of interest. Since many frog and toad species are vulnerable, habitat management strategies like the creation of artificial wetlands or the removal of invasive species-are often a successful practice [7]. Mitigation strategies may include simulating natural forest disturbances or promoting organisms that feed on chytrid fungus [8]. Both these strategies change surrounding ecosystem and consequently, how the amphibians and the fungus interact with their environment and with each other.
For example, from studying common mistfrog (Litoria rheocola) infections both before and after a cyclone, researchers saw that the frog had significantly less infection after the storm [9]. This is thought to be due to the reduced canopy cover of the forest: with less shade came more direct sunlight, more heat, and drier conditions [9,10]. These are all factors that are associated with decreased chytrid spread and virulence [10]. Additionally, the promotion of chytrid-feeding organisms may be another method with less knock-on effects to other species. By introducing/promoting predatory microorganisms, chytrid spore populations can be better managed [11]. Overall, through various ecological management strategies of protected areas, chytrid fungus can be managed to ensure the preservation of vulnerable amphibian species in equatorial peaks.
References
- Weldon, C., du Preez, L. H., Hyatt, A. D., Muller, R., & Spears, R. (2004, December). Origin of the Amphibian Chytrid Fungus. https://www.ncbi.nlm.nih.gov/pmc/articles/PMC3323396/.
- NSW Environment and Heritage. Frog chytrid fungus. (2020, October 14). https://www.environment.nsw.gov.au/topics/animals-and-plants/native-animals/native-animal-facts/frogs/threats-to-frogs/frog-chytrid-fungus.
- Ohio Department of Natural Resources Division of Wildlife. (n.d.). Chytridiomycosis (Amphibian Chytrid Fungus Disease). https://ohiodnr.gov/static/documents/wildlife/wildlife-management/Chytridiomycosis%20Amphibians.pdf
- Zipkin, E. F., & DiRenzo, G. V. (2022). Biodiversity is decimated by the cascading effects of the amphibian-killing chytrid fungus. PLoS Pathogens, 18(7), e1010624. https://doi.org/10.1371/journal.ppat.1010624
- Savage, A. E., Sredl, M. J., & Zamudio, K. R. (2011). Disease dynamics vary spatially and temporally in a North American amphibian. Biological Conservation, 144(6), 1910–1915. https://doi.org/10.1016/j.biocon.2011.03.018
- Sasso, T., McCallum, H., & Grogan, L. (2021). Occurrence of Batrachochytrium dendrobatidis within and between species: A review of influential variables as identified from field studies. Biological Conservation, 262, 109300. https://doi.org/10.1016/j.biocon.2021.109300
- Scroggie, M. P., Preece, K., Nicholson, E., McCarthy, M. A., Parris, K. M., Heard, G. W. (2019). Optimizing habitat management for amphibians: From simple models to complex decisions. Biological Conservation, 236, 60-69. https://doi.org/10.1016/j.biocon.2019.05.022.
- Garner, T. W. J., Schimdt, B. R., Martel, A., Pasmans, F., Muths, E., Cunningham, A. A., Weldon, C., Fisher, M. C., Bosch, J. (2016). Mitigating amphibian chytridiomycoses in nature. Philosophical Transactions B, 371(1709): 20160207. https://doi.org/10.1098%2Frstb.2016.0207
- Roznik, E. A., Sapsford, S. J., Pike, D. A., Schwarzkopf, L., Alford, R. A. (2015). Natural disturbance reduces disease risk in endangered rainforest frog populations. Scientific Reports, 5: 13472. https://doi.org/10.1038%2Fsrep13472.
- Puschendorf, R., Hodgson, L., Alford, R. A., Skerratt, L. F., VanDerWal, J. (2013). Underestimated ranges and overlooked refuges from amphibian chytridiomycosis. Diversity and Distributions, 19(10), 1313-1321. https://doi.org/10.1111/ddi.12091.
- Schmeller, D. S, Blooi, M., Martel, A., Garner, T. W. J., Fisher, M. C., Azemar, F., Clare, F. C., Leclerc, C., Jӓger, L., Guevara-Nieto, M., Loyau, A., Pasmans, F. (2014). Microscopic Aquatic Predators Strongly Affect Infection Dynamics of a Globally Emerged Pathogen. Current Biology, 24(2), 176-180. https://doi.org/10.1016/j.cub.2013.11.032.
Featured Ecologists:
Elzada U. Clover
Clover and her graduate student wanted to know the flora of the Colorado river and its tributaries. As one of the largest and most dangerous bodies of moving water in the US, it presents a number of unique habitats it was attractive both for its likelihood of presenting species both rare and new to science. It is also valuable in helping to understand the movement of plant species along large tracts. Primary to their methodology was the collection of plant specimens, obtaining several hundred, with specimens the nature of their surroundings and the soil or medium they grew in were noted. Each location was also kept insofar as it reasonably could be, and comparisons were made when the journey concluded. There were 4 chief conclusions of the study. 1: There is little vegetational variety, but they are largely undisturbed. 2: While it is a path of migration for lower sonoran species, it is not nearly as much as previously thought. 3: There are relicts of prairie, mesophytic, hydromesophytic, and hydrophytic flora in the canyon, some of which can be explained by a climate similar to that of the Miocene. 4: Altitude, light, moisture, and temperature are more influential in determining species range than geologic data.
Sylvia Earle, Phd
Sylvia Earle revolutionized marine ecology and biology research. She cataloged over 20,000 species of algae and aquatic plants in the Gulf of Mexico using SCUBA. SCUBA was a new technology, so her research was some of the first research studies to use SCUBA. Her research question was what type and distribution of algae are present; her findings provided a vast amount of insight into the ocean landscape. This connects to the concepts of biodiversity and species distribution patterns we’ve discussed in class. Earle has become a major advocate for ocean conservation.
In the 1970s, she also was the lead scientist on the Tektite II Project, which involved scientists living and conducting research 50 feet underwater. (Side note: The government did not want a male and female crew–and would have preferred a male-only crew–but Sylvia Earle was the most experienced diver. So instead, Earle led an all-women team to live and collect photographs of marine life 50 feet underwater for two weeks.) The crew photographed and studied the effects of pollution on coral. In her mid-career, she gave talks around the world about her deep-sea explorations and helped ignite a passion in others for conserving marine life. Dr. Earle eventually became the first woman to be the Chief Scientist of the National Oceanic and Atmospheric Administration (NOAA). She was also named the first Time magazine “Hero of the Planet”. In total, she has spent over 7,000 hours underwater and has authored over 200 papers.
Rosemary Gillespie, Phd
Rosemary Gillespie mainly researches the evolutionary patterns of arthropods on the Pacific islands or archipelagos. Some of her research has included using metabarcoding and machine learning of arthropods, and analyzing arthropod species and population assemblage based on the Hawaii islands’ chronosequence. Gillespie’s research relates to our class material because she is interested in better understanding the drivers of the biological diversity of populations such as geography, invasive species, migration, and trophic levels. Gillespie’s major findings are discovering the various impacts of global change on the biodiversity of arthropod communities. The research performed by Gillespie is important because it broadens our knowledge of how biodiversity changes over time as a result of global change. Arthropod communities are the most diverse macrobiota on Earth and play critical roles in food webs so understanding the changes in their biodiversity is needed. Gillespie supports others to pursue science careers also. She has led many programs that encourage undergraduates to participate in biological research.
Wilnelia Recart Gonzalez
Wilnelia Recart Gonzalez is a Postdoctoral Fellow in the Department of Biology at the University of San Diego. Gonzalez is currently doing research into the interactions between invasive and non-invasive species reproduction and how they are influenced by climate change. Gonzalez started by comparing native plant reproduction in the presence and absence of invasive plant species. She then moved her research into more specific environmental issues caused by climate change. The first issue she tackled was water availability. Gonzalez researched how water availability affects the dynamics between pollinator species and plant reproductive success. Her latest publication is about how pollinator species pathogens are affecting plant reproductive success and adaption. These studies will lay the groundwork for future research into the adaptions caused by climate change and how conservationists can combat these effects. Gonzalez is passionate about diversity in STEM and has been working with middle schools in her area to get students involved in science education. Gonzalez was recently awarded the Commitment to Human Diversity in Ecology Award by the ESA, and she is now recognized for her success in diversifying the future field of ecologists with her outreach, mentoring, and teaching experience.
Ruth Patrick, Phd
Dr. Ruth Patrick was one of the pioneers in studying diatom biodiversity as a way to understand the health of a body of freshwater. She also specialized in understanding the effects of pollution on the health of rivers, lakes, and drinking-water sources by studying diatom response. In 1945, she invented the Catherwood diatometer, an instrument that can collect diatoms, a group of single-celled algae, and was the first to claim that a healthy diatom composition in a body of freshwater should be species-rich and diverse. She then used this to prove that pollution had an effect on diatom composition. She was also the first to identify the health of a river based on plant and animal composition alone, giving rise to what’s known as the Patrick Principle: the belief that biodiversity is the chief indicator of water health. Her research of fossilized diatoms found that the Great Dismal Swamp between North Carolina and Virginia was once a forest, and also that the Great Salt Lake was not always salty. Her research on the health of streams and rivers by monitoring diatom diversity sparked national interest and research in diatom science, not to mention that after volunteering and working for the Academy of Natural Sciences for many years, she became the first woman and the first environmentalist to serve on the DuPont board of directors in the 1950s. She went on to consult Presidents Lyndon B. Johnson and Ronald Reagan on water quality issues such as pollution and acid rain, and earned the National Medal of Science from Bill Clinton in 1966.
Diana Wall
Diana Wall is a soil ecologist who studies soil biodiversity. Her research aims to determine how soil biodiversity contributes to ecosystem services and how climate change will impact this diversity. She conducts her research within Antarctica’s dry valleys, where climate change impacts are amplified to understand these questions. Her interest in soil microbes and nematodes has better uncovered the life histories of species that survive in these harsh conditions. Namely, she discovered Scottnema lindsayae, a soil nematode species, to have a surprisingly broad distribution and high abundance in dry, nutrient-deficient soils. Diana Wall’s research within these habitats can be used to determine how species and ecosystems may respond to the increasing pressures from climate change.
Student contributors:
Note Outline: Paige Fryar, Brooke Burns, Evelyn Rowan, Jakob Fix
Blog Style Summary: Iris Horton, Shannon Dolan, Hannah Werner, Tessa Ferguson
Spotlight on NC: Eli Benbenek, Rebecca Olson, Trey Kaufman
Featured Ecologists: Shannon Dolan, Noel Griffin, S.U., Arden Marsicano, Madison Shrader, Reece Neiderer
How to Apply Ecology in a Changing World: Oliver Roper, Mae Bryant Cates, Abi Finch
the variation within and between different types of life; often quantified as richness (i.e., number of species)
an event in which 75% or more of all species go extinct within a period of 2 million years
diversity index for estimating the total number of species in a population, based on the observed species abundance in a given sample
a method of plotting the total number of different species have been encountered in a given number of samples, that helps ecologists estimate the species richness in a sample or community of interest, and to gauge how much sampling is “enough” to get an accurate picture of the community
biodiversity tends to be highest at/around the equator; this pattern is true for both terrestrial and aquatic species
areas where biodiversity decreases, particularly in desert areas