Chapter 8: Parasitism and Mutualism
Vocabulary:
- Coevolution: perpetual adaptation to another organism
- Density-dependent transmission: transmission increases with the number of hosts in a given area
- Ectoparasites: parasites that live on or in the skin but not inside the host’s body (Ex: ticks, fleas, lice).
- Endoparasites: live inside the host organism (Ex: GI tract, lungs, liver, roots).
- Episodic feeders: do not interact with their host for long enough to be considered parasitic (vampire bats and mosquitoes are examples).
- Frequency-dependent transmission: reliant on a number of interactions with hosts
- Kleptoparasitism: “parasitism” by theft (not true parasitism). Kleptoparasites steal food (e.g., prey) from another animal as their main feeding strategy, but do not feed directly from a host’s body.
- Brood parasitism: a specific type of kleptoparasitism; a reproductive strategy characterized by one species laying their eggs (parasite) into the nest of another species (host). Brood parasites steal food and energy in the form of parental investment from hosts
- Macroparasites: visible to the naked eye. Spend a longer amount of time with the host compared to the host’s lifespan.
- Microparasites: microscopic parasites. Generally manifest as a disease and lasts a relatively short time compared to the host lifespan.
- Ex: Viruses and bacteria
- Note that microparasites are different from pathogens, which cause disease but do not associate with the host for extended periods of time
- Mutualism: both species benefit from the relationship
- Parasite: an organism that takes nutrients and energy from another living organism (called the host), but does not kill them. Parasites typically associate with their host for an extended period of time.
Question from a Student:
“How can we tell a true parasite from a parasitoid or an episodic feeder?”
Answer: Great question!
1. True parasites such as the porcine tapeworm (Taenia solium) have long-term, close relationships with their hosts. They are often with their hosts for their entire life cycle and complete multiple stages of life. For this reason, they do not typically kill their hosts. 2. Parasitoids such as Cotesia congregata usually spend only their egg and larval stages with their hosts and will ultimately kill them. 3. Episodic feeders such as leeches (i.e., Hirudinea verbana) have short-term, encounter relationships with their hosts and will leave them once satiated. They can be from any life stage.
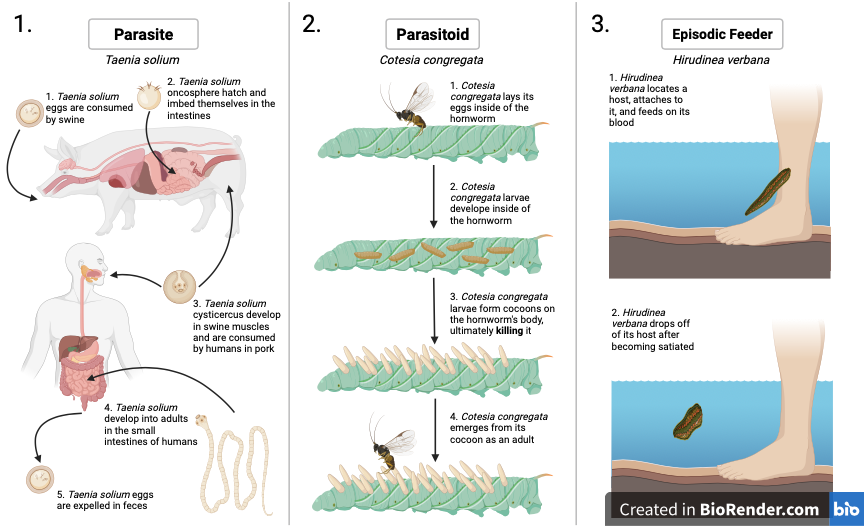
Outline of Notes:
Requirements for Parasitism:
True Parasites take nutrients and energy from another living organism (host), but do not kill them. They typically associate with their host for an extended period of time.
- Requirements for interaction to be considered parasitism:
- Occurs between different species
- Long term relationship
- an encounter (Ex: Vampire bats, mosquitos) is most likely an episodic feeder, not a true parasite.
- Requirements for an organism to be considered a parasite:
- Parasite must be smaller than the host
- Can not sustain itself on an animal smaller than it is
- Specific traits coevolved with host
- Ex: variable pigmentation of lice found on different species of pigeons: pigeons with darker coloration have darker lice
- High reproductive rate and high offspring numbers compared to host
- Kleptoparasitism is parasitism by theft, and is not considered true parasitism
- Ex: Cowbirds lay their eggs in the nests of other birds. When the cowbird chick hatches, it pushes the host’s eggs out of the nest and steals parental care from any other chicks that manage to hatch.
Classifications of Parasites:
- Obligate vs facultative:
- Obligate: cannot complete its life cycle without exploiting a suitable host
- Facultative: Resorts to parasitic activity, but does not rely on host to complete life cycle. (Ex: many species of fungi only infect a plant host under specific stressful conditions.)
- Specialist vs generalist:
- Specialist: parasite whose life history evolved to a specific host
- Generalist: a parasite whose life history adapts to many hosts
- Size:
- Microparasites: Need a microscope to identify. Generally manifest as a disease and infection is short compared to the host’s lifespan.
- Ex: protists, protozoa, viruses, bacteria
- Microparasites: Need a microscope to identify. Generally manifest as a disease and infection is short compared to the host’s lifespan.
- Macroparasites: Visible to the naked eye. Long generation time and typically don’t complete a life cycle in the host.
- Ex: flatworms, roundworms, lice, fleas, ticks, zombie fungi (Cordyceps)
- Habitat:
- Endoparasites: reside inside the host’s body, such as in the GI tract, lungs, liver, or roots.
- Ectoparasites: reside on or in the host’s skin, but not inside the host’s body.
- There are advantages and disadvantages to each approach:
|
Advantages (+): |
Disadvantages (-): |
Endoparasitism: |
– Reliable feeding – Protected from the environment and enemies (save energy) |
– Dispersal is more difficult – Vulnerable to the host’s immune system |
Ectoparasitism: |
– Easy dispersal – Safe from the host’s immune system |
– Feeding is more difficult – Vulnerable to the environment and enemies
|
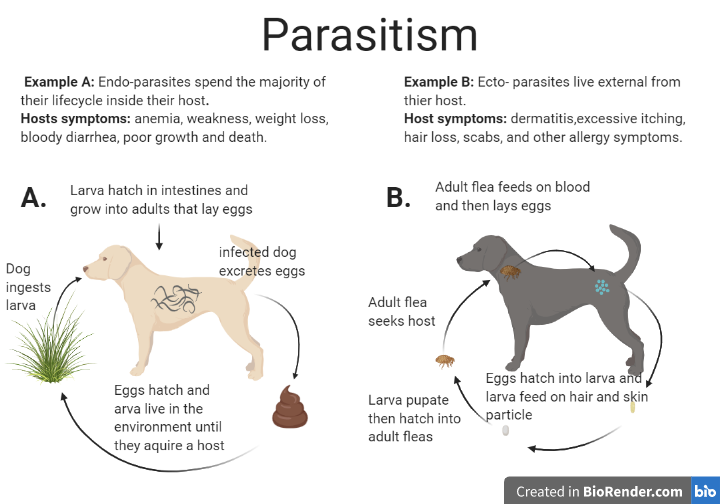
Transmission:
- Transmission through different life stages is important in the transfer and reproduction of parasites.
- A parasite may be a larva in one host species and an active adult in another
- The more complex a parasite’s life cycle, the more likely it is to go extinct
- Direct transmission is safer than indirect transmission for the parasite
- Density and frequency dependence can also play a role in how likely a parasite is to become extinct:
- Density-dependent transmission: reliant on the density of hosts for transmission
- Parasite declines as host populations decline
- Frequency-dependent transmission: relies on the number of interactions with hosts
- transmission through vectors (e.g., mosquito, tick)
- May be able to survive a decline in host populations, as long as there are enough vectors to move between hosts.
- Density-dependent transmission: reliant on the density of hosts for transmission
Host Response to Parasitism:
- Parasites can affect their host’s behavior to achieve goals like reproduction:
- Infected hosts often have less resources for other processes like reproduction, survival, etc.
- This makes it easy for the host to be eaten, which may be necessary for the parasite to continue its life cycle
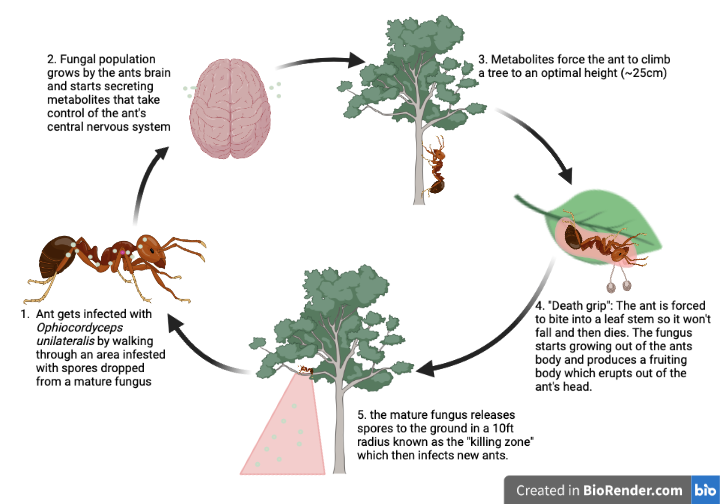
- Host defenses against parasitism:
- Social grooming behaviors (only effective against ectoparasites)
- Internal reactions (inflammation and cysts) can wall off parasites or help expel them.
- Parasites are often able to pacify the host’s immune response through deactivating immune cells → “immune-calming” effects may be beneficial to both host and parasite
Mutualism:
Mutualistic relationships are those in which both species benefit from the interaction.
- Mutualisms involve diverse species interactions and can be involved in the transfer of nutrients.

- Mutualism has trade-offs, especially for endosymbionts
- Ex: many endosymbionts evolve smaller genomes compared to free-living organisms, which makes them more dependent on their host.
- Defensive mutualism:
- One partner receives food and shelter and in return, helps the partner by defending against herbivores/predators/parasites

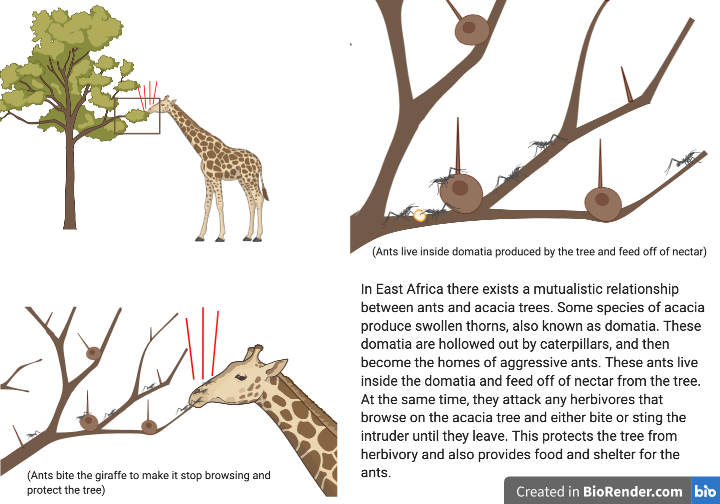
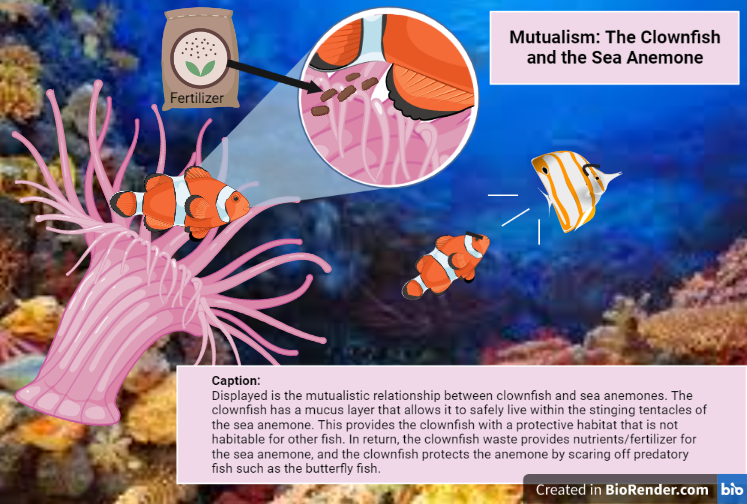
- Symbiosis is context dependent
- Ex: Oxpeckers clean mites off of large herbivores, providing a benefit for the herbivore. However, if there are no mites present, the birds will begin to peck off flesh.

Key Takeaways
Overarching Themes and Unifying Concepts:
- Co-evolution occurs between any two species that are closely associated over many generations.
- The characteristics of a parasite or mutualist evolve in response to their specific host/partner.
- Parasitism and mutualism exist on a spectrum; the nature of a particular relationship between two partners is context-dependent.
- Parasites are not always bad; some hosts may even derive benefits from parasites.
How might this information be applied to address grand challenges?
- Knowing the characteristics of a host can identify potential niches that a parasite might be able to exploit. This would also indicate a potential weakness within the parasite’s life cycle that could be exploited to eradicate them from the host.
- Can we use parasites to improve human health?
Blog-Style Summary:
What is a parasite and why do they have a negative connotation? A true parasite is an organism that has coevolved with their hosts in order to derive nutrients from them. Coevolution is when two or more closely associated species affect each other’s evolution and adaptations. Many people have the common misconception that parasitism equates to death, when that is not necessarily the case. Many parasites need their host to be alive and in decent health in order to survive. However, parasitism is often categorized as a negative species interaction when compared to other types of interactions. Another type of species interaction is mutualism. Mutualism is often described as the inverse of parasitism; it is a positive interaction in which both species benefit.
Being a parasitic organism can have its advantages- some organisms that can survive without being parasitic resort to parasitism because of the benefits, these organisms are called facultative parasites. Other parasites have evolved to the extent that they are not able to survive without exploiting a host, and these organisms are called obligate parasites. Facultative and obligate parasites can be further identified by where they live. Organisms that live within another organism are called endoparasites and organisms that live on the outside of another organism are called ectoparasites. Living within another organism has many benefits such as having a reliable food source and protection from natural enemies and the external environment. However, one downside of being an endoparasite is that it is not easy to disperse. Whereas ectoparasites have the inverse of endoparasites in terms of benefits and disadvantages. Ectoparasites are able to disperse easily and are not directly affected by the host’s immune system. But, they do not have a constant food source and they are exposed to the surrounding environment.
Parasites also come in all shapes and sizes. Macroparasites can be seen by the naked eye while microparasites cannot be seen without a microscope. No matter what size the parasite is, it is vital that the parasite is smaller than its host. They must be smaller than their host to thrive and successfully reproduce. Parasites also must be able to reproduce faster and in greater quantities than their host. Size is one of the ways that scientists are able to identify and classify parasites. Parasites are also classified by their habitat (endo- or ectoparasite), growth location, and host specificity; and plant parasites can be classified based on their photosynthetic abilities.
Some parasites have multiple different hosts (generalists), whereas others have a species-specific host (specialists). Generalists are capable of infecting multiple species, this makes them more adapted to survive factors that reduce host population sizes. Specialists are more likely to go extinct if something happens to their host species’ population. Parasites with more complex life cycles and multiple hosts per life stage are also more likely to become extinct.
The distinction between parasitism and mutualism is not always as straightforward as the common observer might perceive it to be. The relationships that lie in this murky intersection are often some of the most interesting examples of symbiosis in the realm of ecology. Cleaning symbiosis is an excellent example of this intersection. Many are aware of the relationship between the oxpecker and the bison where the oxpecker, a small bird species, will sit on the back of its host and eat irritating and dangerous parasites off of the bison in exchange for a ride and a snack. It is less commonly discussed how the oxpecker will more than happily peck at the open or healing wounds of its host in order to receive its favorite snack of mammalian blood.
Cheating is the term used by ecologists to describe this sort of behavior where a species participating in a seemingly mutualistic relationship will alter its behavior to contrive more of a benefit for itself at the expense of its partner. Cheating behavior reminds us that species involved in any symbiotic relationship, including mutualism, are acting strictly within the confines of their own self-interest. The bacteria in our gut are not concerned with our well-being past the point at which it allows them to reproduce and certainly does not aid in our digestion or fight off viruses because they care for us.
I believe that a main factor that puts us at a disadvantage when it comes to studying symbiotic relationships is our tendency to anthropomorphize the natural world around us. We see these incredibly complex relationships in nature that have evolved over thousands of years and we attempt to assign human values to them and understand them through our own relationships with each other. We see the oxpecker kindly pluck a tick off the bison’s back and think to ourselves how nice it is of the oxpecker to care for the bison in such a manner, then when the oxpecker turns around and digs its beak into the flesh of the bison we almost feel a sense of betrayal from its behavior as if it were a friend who had just broken a long-held promise. All of this is to say that the constant bending spectrum between mutualism and parasitism is a relationship, similar to nearly every other aspect of nature, that is so complex viewing it strictly through a human lens would be to do it a great disservice.
Spotlight on NC:
Parasitism
Monarch butterflies (Danaus plexippus) are a well-known species of butterfly native to North Carolina. They are famous for their bright orange coloration and epic migrations. What most people don’t know is that these butterflies and their close relatives the queen butterflies (Danaus gilippus) are the only hosts for a parasitic protozoan that has coevolved with them called Ophryocystis elektroscirrha (OE). These true parasites are obligate, meaning that they rely on their host in order to complete their life cycle. Without their host, they would be unable to reproduce and would die off. They are endoparasites that live inside of their hosts for the majority of their life cycle (Fig.1).
OE spores lay on the butterfly’s cuticle between the scales that cover their body and wings (Fig.2) and are scattered onto milkweed plants when females lay their eggs or others feed on the milkweed. These spores are then ingested by monarch caterpillars when they hatch from those eggs and begin eating the surrounding leaves. This is an example of vertical transmission, when adults pass parasites on to their offspring. Once inside the caterpillar’s digestive system, the spores begin to open and the parasites move towards the cells that produce the cuticle. When the caterpillar pupates the protozoans sexually reproduce, creating new spores that will coat the new adult butterfly and begin the cycle again [1].
This relationship is harmful to the butterflies OE relies on for its life cycle. Infected individuals have lower survival rates. These effects are worsened when larvae ingest spores earlier in their development. Infected individuals have smaller body sizes and mate less than healthy individuals [2].
OE is present in both the eastern and western migratory populations of monarch butterflies in North America. The Western population has a higher proportion of OE-infected individuals (30%) than the Eastern population (8%) [4]. However, those numbers could be rising soon in the eastern population due to the influence of helpful gardeners trying to support the monarchs by planting Asclepias curassavica, also known as tropical milkweed.
The long-distance migration of monarch butterflies is essential in keeping levels of OE from overwhelming the population through two mechanisms: migratory escape and migratory culling. As monarchs make their yearly journey between their northern summer breeding grounds to their southern overwintering sites in central Mexico, they escape areas that have been contaminated by OE and enter fresh sites to reproduce in, preventing a build-up of spores that could result in more severe infection [1]. Migratory culling also results in decreased parasite prevalence in the overwintering sites as monarchs weakened by infection cannot fly as far, limiting the spread of OE [1].
Behavioral changes in the migrational patterns of monarchs directly affect parasite transmission. Tropical milkweed is not native to the United States, and unlike our native milkweeds, it does not die back over winter in warm climates like those found in the southeastern USA, but continues to grow foliage and flower throughout the fall and winter [5]. The dying off of milkweed in fall is one of the signals for monarch butterflies to enter reproductive diapause and return to their overwintering sites [6]. The presence of milkweed patches that are still green and usable for breeding influences some monarchs to remain reproductively active throughout the winter [6]. This phenomenon of winter breeding sites has been found in many southeastern states, as far north as North Carolina and Virginia [7]. OE levels in these winter breeding sites are far higher than in migratory populations due to the lack of migratory escape and migratory culling, and migratory monarchs that pass through these areas are also subjected to an increased risk of transmission from resident monarchs [6]. This demonstrates how the life cycles of hosts and obligate parasites are intricately intertwined, and behavioral changes in the host can directly affect the transmission and population size of the parasite. It is also an example of how humans acting with good intentions can sometimes have negative consequences in natural ecosystems.
Mutualism
Unlike parasitism, not every species interaction has a clear winner and loser. Mutualism is a relationship between two or more species in which both participating species benefit from the presence of the other. This is often confused with cooperation, which is when two organisms of the same species benefit from their relationship with one another [8]. In some cases of mutualism, partner species are so dependent on each other that they literally cannot survive without the other. Those cases would be described as obligate mutualism. An example of obligate mutualists are termites and the protozoa that live in their digestive tract. The protozoa need the termite’s digestive tract as a habitat and place to obtain food while the termites require the protozoa in order to digest the wood they eat [9]. On the contrary, facultative mutualism describes a mutualistic relationship where the species benefits from the interaction with the other species but does not require the relationship for survival. Humans’ and cats’ relationship would be considered facultative mutualism. Although humans derive great joy, pest control and companionship from cats and cats derive food, clean water, and shelter from humans, both species can survive just fine without the other [10]. Mutualism is often categorized as defensive or dispersive. Defensive mutualism is when one species receives something such as food or shelter from the other and in turn, provides defense and protection. Dispersive mutualism is when one species gains food from the other and in turn may help with the dispersal of pollen or even seeds. The following section involves an example of a dispersive mutualistic relationship.
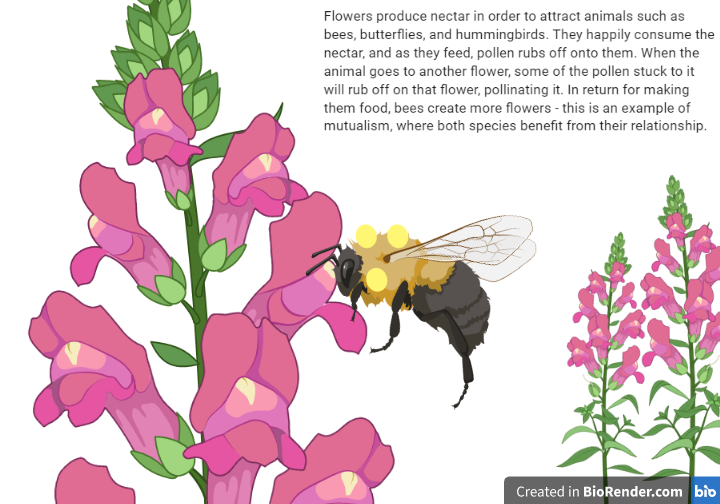
with milkweed plants wherein both species benefit [12,13,14]. First, monarchs visit milkweed plants in search of food. They consume the plant’s nectar, which is rich in sugar and nutrients. As they forage, the butterflies unintentionally collect pollen on their bodies. This pollen is then transferred from one milkweed plant to another as the monarchs continue to find food. The butterflies benefit from drinking nectar, while the milkweed plants benefit from pollen dispersal, and successful pollination guarantees future generations of milkweed [12].
in the media today because of its importance in terrestrial ecosystems. Within North Carolina, monarch butterflies have a crucial symbiotic relationship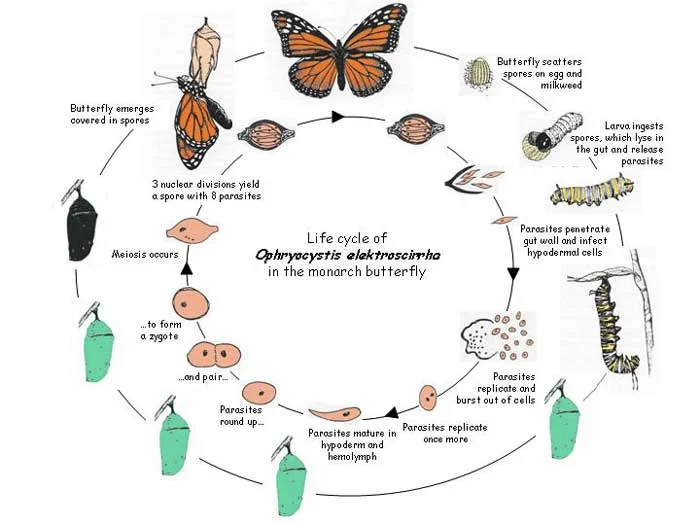
In addition to pollination, milkweed also plays a vital role in the monarch butterfly life cycle [13]. Female monarchs rely solely on milkweed plants for reproduction because they lay their eggs on the underside of the leaves. These leaves contain poisonous chemicals called cardiac glycosides that deter predators from consuming the plant. However, monarch butterflies have coevolved with milkweed so that they are not affected by the toxins [12]. Once the eggs hatch, the young caterpillars are able to dine on the leaves without getting sick. The chemicals accumulate in the tissues of the developing larva and persist through metamorphosis into adulthood [13,14]. Thus, monarch butterflies have bright coloration patterns to warn predators that they contain poisonous toxins and should not be consumed. The monarch-milkweed relationship is an excellent example of coevolution wherein both species evolved in response to the other, and this relationship is illustrated in Figure 12. Unfortunately, monarch butterflies are now considered an endangered species. The loss of milkweed plants and suitable habitats due to climate change is threatening their survival [14], especially in North Carolina. These pollinators are a top priority for wildlife scientists in NC as they work to preserve and protect this delicate mutualism [13].
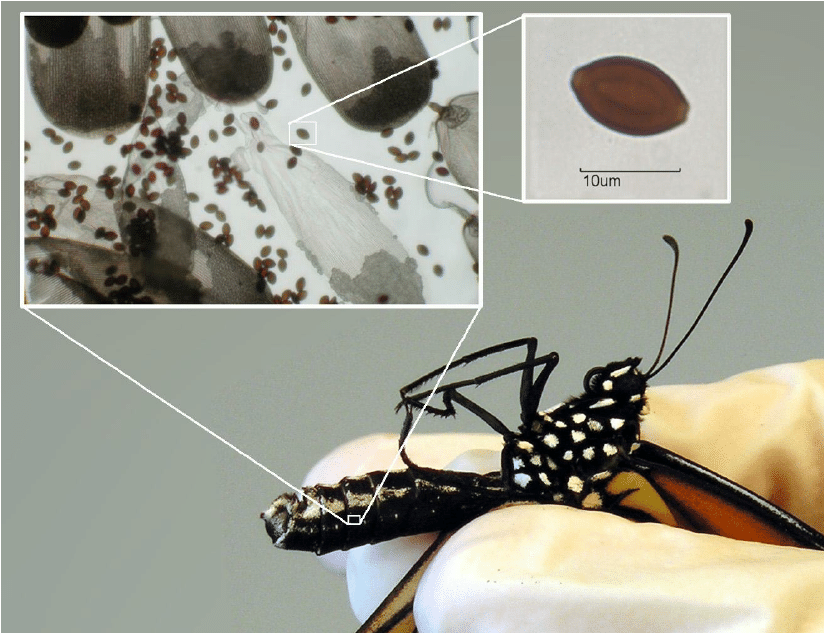
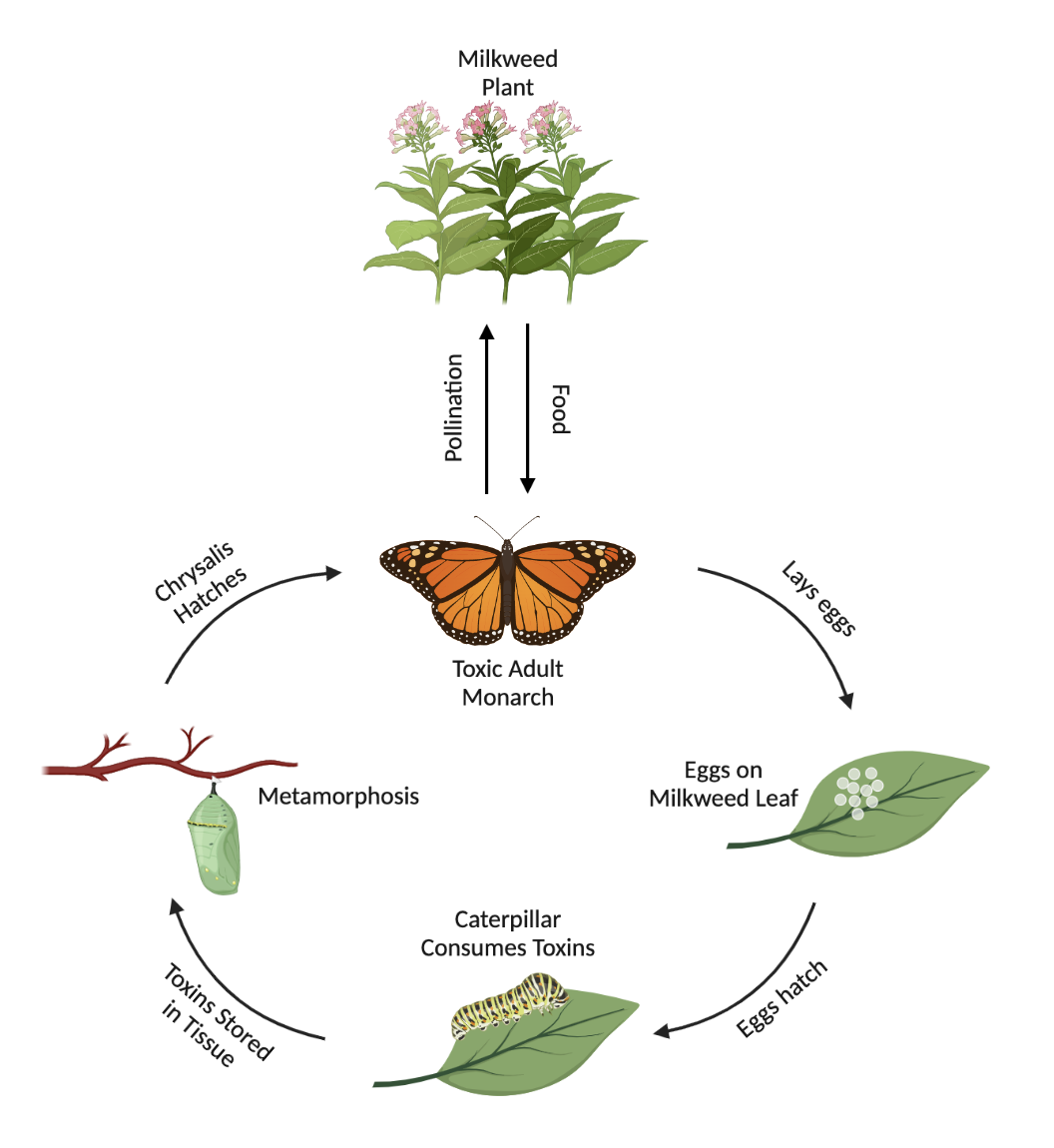
How to Apply Ecology in a Changing World:
Save the… Parasites?!
Despite excitement about the prospect of eliminating the pests that prey on humans, parasites, organisms that live on (ectoparasite) or in (endoparasite) a host organism, are vital for a healthy ecosystem and should be preserved for their role [1]. Parasitic organisms coevolved with their more charismatic hosts, and climate change is testing the strength of these evolutionary bonds [2]. Parasites are valuable links in the food chain and can serve as indicators of a healthy ecosystem. Their loss can have shockwave effects that are gaining more attention as climate change endangers the host species or creates inhospitable environments for the parasites [3]. Some studies have found that a higher diversity of parasites can be a buffer against dangerous pathogens [1]. However, parasites can still be a difficult topic for conservation research. This is because they have an economic and/or health cost to human and wildlife populations.
Parasites that are specialists and closely rely on one to two specific host species are most at risk, while generalists can hop between species and hedge their bets on success. Similarly, parasites that require multiple hosts to complete their life cycle are more likely to face extinction. Parasitic species will have a shorter reproductive cycle than their host species and typically have a large number of offspring to better ensure their survival.

Conservation initiatives are only beginning to recognize the importance of retaining the parasitic species along with the hosts we feel more strongly about, such as whales and bees [5]. While it’s difficult to garner support for protecting nematodes and tapeworms, their conservation programs will lurk just under the surface as they continue to allow their ecosystems to flourish. One such parasite that has beneficial effects on its environment is a Japanese nematomorph that infects small arthropods, such as crickets, and causes them to jump into bodies of water where the parasite can then reproduce. This may sound detrimental to the arthropod communities, but it is actually a natural form of population control. Additionally, these crickets that jump into the water have been found to provide up to 60% of the endangered Japanese char’s annual energy intake [8]. Without these nematomorphs, an already endangered species would lose a significant portion of their diet making their survival even more difficult than it already is. In the wake of the continuing threats faced by parasite species and the misunderstood importance of their role in the environment, a group of scientists have come up with a 12 step global parasite conservation plan [9]. These 12 goals are grouped into the following themes: data collection and synthesis, risk assessment and prioritization, conservation practice, and outreach and education. These scientists want to better understand the world of parasites in order to better protect them and change historical understandings about their importance. They even go so far as to propose the lofty goal of describing at least half of the parasite diversity on this planet. While the general public tends to think of parasites as harmful to life on earth, many scientists are working really hard to rewrite this narrative and work towards parasite conservation instead.

Gaining support for the conservation of parasitic species will require changing the narrative around these species. If we continue to view them as non-beneficial/harmful then garnering support will be difficult. However, if we can show people the benefits of parasitics then maybe we can change the narrative. For example, helminths have been shown to be a great alternative to conventional medical treatments for autoimmune disorders, inflammatory diseases, and neuropsychiatric dysfunctions [6]. Patients who used helminths as opposed to conventional medical treatments reported little to no side effects, higher reports of effectiveness/satisfaction, and fewer residual effects of the condition after treatment. These results make the use of Helminths a surprisingly favorable option when compared to conventional medical treatment. Parasites will build up your immune system in order to fight off other pathogens while keeping your immune system weak enough so it does not expel them [7]. This all goes back to the idea that the presence of parasitic species indicates a healthy ecosystem. If we can drive home the point that parasites can be beneficial and border a mutualistic relationship then garnering support for conservation efforts will be a lot easier.
References
- Dougherty, E. R., Carlson, C. J., Bueno, V. M., Burgio, K. R., Cizauskas, C. A., Clements, C. F., Seidel, D. P., & Harris, N. C. (2015). Paradigms for parasite conservation. Conservation Biology, 30(4), 724–733. https://doi.org/10.1111/cobi.12634
- Carlson, C. J., Burgio, K. R., Dougherty, E. R., Phillips, A. J., Bueno, V. M., Clements, C. F., Castaldo, G., Dallas, T. A., Cizauskas, C. A., Cumming, G. S., Doña, J., Harris, N. C., Jovani, R., Mironov, S., Muellerklein, O. C., Proctor, H. C., & Getz, W. M. (2017). Parasite biodiversity faces extinction and redistribution in a changing climate. Science Advances, 3(9), e1602422. https://doi.org/10.1126/sciadv.1602422
- Panko, B. (2017, September 7). The World’s Parasites Are Going Extinct. Here’s Why That’s a Bad Thing. Smithsonian; Smithsonian.com. https://www.smithsonianmag.com/science-nature/parasites-are-going-extinct-heres-why-thats-a-bad-thing-180964808/
- Cizauskas, C. A., Carlson, C. J., Burgio, K. R., Clements, C. F., Dougherty, E. R., Harris, N. C., & Phillips, A. J. (2017). Parasite vulnerability to climate change: An evidence-based functional trait approach. Royal Society Open Science, 4(1), 160535. https://doi.org/10.1098/rsos.160535
- Sommer, L. (2020, August 7). Scientists Say Many Parasite Species Are At Risk Of Extinction And Need Protection. NPR.org. https://www.npr.org/2020/08/07/900350897/scientists-say-many-parasite-species-are-at-risk-of-extinction-and-need-protecti
- McKenney, E. A., Williamson, L., Yoder, A. D., Rawls, J. F., Bilbo, S. D., & Parker, W. (2015). Alteration of the rat cecal microbiome during colonization with the helminth Hymenolepis diminuta. Gut Microbes, 6(3), 182–193. https://doi.org/10.1080/19490976.2015.1047128
- Cheng, A. M., Jaint, D., Thomas, S., Wilson, J. K., & Parker, W. (2015). Overcoming Evolutionary Mismatch by Self-Treatment with Helminths: Current Practices and Experience. Journal of Evolutionary Medicine, 3, 1–22. https://doi.org/10.4303/jem/235910
- Sato, T., Watanabe, K., Kanaiwa, M., Niizuma, Y., Harada, Y., & Lafferty, K. D. (2011). Nematomorph parasites drive energy flow through a riparian ecosystem. Ecology, 92(1), 201–207. https://doi.org/10.1890/09-1565.1
- Carlson, C. J., Hopkins, S., Bell, K. C., Doña, J., Godfrey, S. S., Kwak, M. L., Lafferty, K. D., Moir, M. L., Speer, K. A., Strona, G., Torchin, M., & Wood, C. L. (2020). A global parasite conservation plan. Biological Conservation, 250, 108596. https://doi.org/10.1016/j.biocon.2020.108596
Featured Ecologists:
Sonia Altizer, PhD
Sonia Altizer is a professor at the Odum School of Ecology at the University of Georgia. She runs the Altizer lab that focuses broadly on the ecology of infectious diseases, coevolution between hosts and parasites, and insect-pathogen interactions. Altizer has a number of different research projects that she works on with UGA graduate students. A majority of her work involves monarch butterflies, and she helps run a citizen science project called Monarch Health which monitors infectious diseases in wild monarch populations. In addition to butterflies, Altizer also studies the migration patterns of birds, marine and terrestrial mammals, and other insects. Her lab places a lot of emphasis on the impacts of anthropogenic change on infectious diseases and the ways in which human behavior affects disease dynamics. One of her previous projects, which looked at geographic variation in monarchs, used molecular genetic techniques to understand phenotypic variation between different populations based on regional migration and geographic patterns. Altizer’s research on animal migration systems and infectious disease ecology has important implications for future conservation and management strategies.
Priyadarshini Chakrabarti Basu, PhD
Dr. Priyadarshini Chakrabarti Basu studies pollinator health with a focus on mitigating stressors to bee health. Her research facility, the Chakrabarti-Basu Bee Lab at MSU, looks at stressors such as climate change, pesticides, and poor nutrition in particular. Dr. Chakrabarti-Basu also discovered specific impairments that pesticides can cause in bees. Previously, she worked as a postdoctoral fellow at Dr. Ramesh Sagili’s Honey Bee Lab at Oregon State University, where she mapped pollen diversity. She has found that malnutrition is one of the biggest causes of global bee pollinator declines and that sufficient pollen diversity is vital for their health. Dr. Chakrabarti-Basu’s research has implications for keeping pollination systems in balance and preventing further ecological harm. It may also change human behavior, as landscapers may become more knowledgeable regarding what pesticides have extreme adverse effects and what plants are nutritionally beneficial for pollinators. Topics that connect to our class discussions include the viability of parasites and pathogens, mutualistic pollen-pollinator relationships, gut microbiomes and nutritional qualities, survival and fitness, and more. She is also extremely passionate about mentoring undergraduate and graduate students and believes that indigenous and local knowledge is vital to protecting biodiversity.
Melissa A. Miller
Dr. Miller primarily researches invasive and foreign species in the Everglades of Florida. She researches the impacts of introduced pathogens and parasites from invasive species. Lots of research has been done on the direct impacts that burmese pythons, or other invasive species, have on local species. Dr. Miller has researched the impact that parasites introduced by burmese pythons have had on local reptile species. Her research has found that one parasite in particular, Raillietiella orientalis, has spread from the introduced pythons to native snake species. R. orientalis is a parasitic crustacean that primarily lives within the lungs and airways of snake species. This species spreads when the eggs are defecated by the python, end up in a frog or lizard from the environment, then that frog or lizard is consumed by a native snake. Native snake species that have been found with these parasites include corn snakes, black racers, coachwhip, cottonmouth, and several other species. Dr. Miller’s research has shown that this parasite has even spread beyond the range of introduced pythons. Dr. Miller has also done research on parasites from tegus, as well as other invasives, in the Everglades. This research has shown the importance of studying invasive species beyond the direct impacts.
Amy B. Pedersen
Dr. Pedersen studies diseases ecology and evolution, she works to understand real world implications of co-parasites and parasites that can infect multiple hosts. She conducts research on wild mice communities to better understand the roles of co-infections and the strategies of treatment to apply to global health issues. Dr. Pedersen’s research has found that it is very frequent and are more detrimental for a host organism to have multiple parasites infecting it at once. Yet, current parasite research methodology mostly focuses on a single parasite at a time disregarding the interactions multiple parasites may have upon their shared host. The problem with looking at co-infecting parasites is that the current methods to look at impacts of parasites only focus on a single parasite not applying these finding to real world situations where co-parasites are very common. Due to this Dr. Pedersen suggests using community ecology methodologies to determine the impacts of co-infecting parasites. Along with determining how much co-parasites affect organisms with the multi-system infections (such as I show in my BioRender) as this has been found to disproportionately impact the host organisms.
Student Contributors:
Note Outline: Abby White, Taylor Pack, Chase Edmondson, David Holden
Blog Style Summary: Lauryn Bainbridge, Jordan Reimers, Lily Zeller, Ryan Hamilton, Cameron Downing
Spotlight on NC: Vanessa Schaefer, Dorian Hayes, E Redick, Lindsey Wilson
How to Apply Ecology in a Changing World: Ceridwyn Brown, Arden Marsicano, Jake Johnson, Lauren Stanfa
Featured Ecologists: Lindsey Wilson, Ren Rooney
an organism that takes nutrients and energy from another living organism (called the host), but does not kill them. Parasites typically associate with their host for an extended period of time.
perpetual adaptation (in response) to another organism
both species benefit from the relationship
parasites that live inside the host organism (e.g., internal habitats such as gastrointestinal tract, lungs, liver, roots)
parasites that live on or in the skin (e.g., external host habitat), but not within the body
parasites that are visible to the naked eye. Macroparasites spend a longer amount of time with the host in proportion to the host’s lifespan.
microscopic parasites. Generally manifest as disease and last a relatively short time compared to host lifespan (e.g., viruses, bacteria)