Chapter 9: Community Ecology
Vocabulary:
- Community: a group of populations of different species that occupy the same area within a boundary and interact directly or indirectly
- Assemblage: “a taxonomically related group of species that occur together in space and time” (Stroud et al., 2015)
- ex: a lowland forest amphibian assemblage
- Association: a type of community with relatively consistent species composition, uniform general appearance, and distribution that is characteristic of a particular habitat (i.e. oak-hickory forest); adapted to specific environmental selective pressures
- Climax community: a relatively stable community that forms over a long period of time without major disturbances, whose membership includes species that are adapted to the stressors associated with their given environment
- Intermediate community: transitional stage of succession characterized by higher turnover of generalist and competitive species
- Assemblage: “a taxonomically related group of species that occur together in space and time” (Stroud et al., 2015)
- Disturbance: an event that disrupts a community and clears or alters some niches (e.g., hurricane, wildfire)
- Diversity: the variety and/or variability of life in a given area
- Alpha diversity: a metric to quantify the diversity of types of organisms and/or their relative abundance
- Beta diversity: variation between communities, resulting either from differences in membership (which species are present / absent) or in the relative abundance of species
- Gamma diversity: the overall species diversity across communities within a larger geographic area
- Evenness: a measure of alpha diversity that quantifies the extent to which species in a community have similar relative abundance
- Guild: a group of species that use similar resources in similar ways
- Ex: insects that collect nectar in similar ways, predators that consume similar prey
- Keystone species: a species that has a disproportionate effect on the rest of the community. (Keystone species may not be the most abundant, but they have a tremendous impact on other species and the broader environment.)
- Pioneer species: highly competitive and opportunistic (sometimes weedy) species that establish themselves during primary succession and may make the habitat more hospitable (i.e. nitrogen fixation, changing pH levels)
- Rank abundance: species are listed in order of decreasing relative abundance in a community, with the most abundant species listed first
- Relative abundance: the proportion of individuals in a community that belong to a particular species. The relative abundance of all species in a community add up to equal 1.
- Richness: a measure of alpha diversity that quantifies the number of species in a community
- Succession: predictable patterns in the formation of communities.
- Stages of succession:
- primary succession – featuring generalist and opportunistic species
- intermediate succession – featuring competitive species
- climax communities – featuring species adapted to specific stressors of a particular environment
- Stages of succession:
- Vertical structure: how biodiversity varies along a vertical gradient.
- Zonation: spatial change in community structure; relates to structure and species composition
Question from a Student:
“Does anyone have a mental tool or some strategy to remember the differences between alpha, beta, and gamma diversity? I’ve “learned” them 3-4 times in the last year and always need a reminder for at least one.”
Answer, from Ceridwyn Brown: I use alpha diversity occurs in a single place, while beta diversity occurs between places, and gamma diversity is on a geographical scale. I hope that helps!
Outline of Notes:
Community Ecology:
A community is a group of populations of different species that occupy the same area. These populations interact either directly or indirectly.
- Community and physical structure are context dependent
- Aspects of a community include:
- Number of species
- Relative abundance of each species
- Species interactions
- Physical structure of the environment
Measuring Community Composition:
- Rank abundance: species ranked by relative abundance in the community; the most abundant species are listed first (figure 1)
- On a ranked abundance scale:
- Y-axis: relative abundance of the species
- X-axis: different species in a given community at a given point in time, in decreasing order of relative abundance
- A flat line represents the most even community possible (all species in a community have a similar relative abundance)
- On a ranked abundance scale:

- Most communities are comprised of a few very common species that occur in high densities, and many rare species that occur at very low densities
- Keystone species: a species that has a disproportionate effect on the structure of the rest of the community.
- Ex: Elephant browsing behaviors limit tree growth. Reduced tree growth allows grasses to be favored, thereby forming and maintaining a savanna habitat.
- Ex: Pacific sea otters affect the kelp bed abundance by controlling sea urchin populations. When sea otter populations are low, urchin populations are high and overgraze kelp beds, destroying habitat for other species.
- → Note that different keystone species may incur top-down and/or bottom-up control – and that both types of control may occur simulataneously.
Changes in Communities:
- Communities can change according to temporal patterns.
- Ex: over the course of the day the most common species of zooplankton in the upper layer of a lake will migrate towards the bottom, leaving another zooplankton species that does not migrate to be the dominant species.
- Ex: bird communities change seasonally with migration.
- Vertical structure: how biodiversity is fostered along a vertical gradient. Affects microclimate conditions and community membership.
- Ex: vegetation in a forest shifts from grass and herbaceous ground cover on the forest floor, to shrub and herb layers, to trees that make up the understory and finally the canopy. In a forest, these layers are influenced and determined by the vertical gradient of light.
- Ex: photic and aphotic layers in aquatic ecosystems
- Zonation: spatial change in community structure
- Can be caused by change in elevation (shown in Figure 3)
- Specialists tend to prosper at higher elevations possibly due to adaptations relating to exposure or rockier soil composition
- Often characterized by differing producer communities in response to some environmental gradient
- Ex: different plant species along a salinity gradient or changes in water levels in a salt marsh
- There is not always a clear distinction between zones; it may present itself as a subtle gradient
- Can be caused by change in elevation (shown in Figure 3)
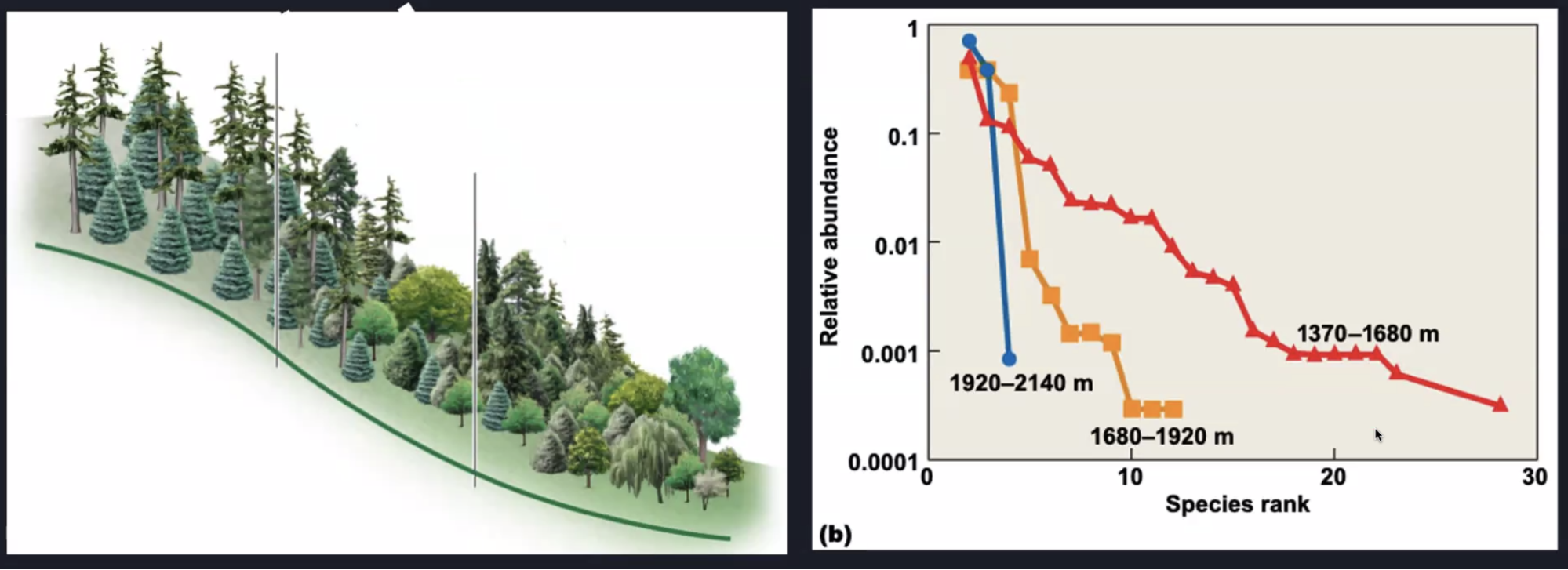
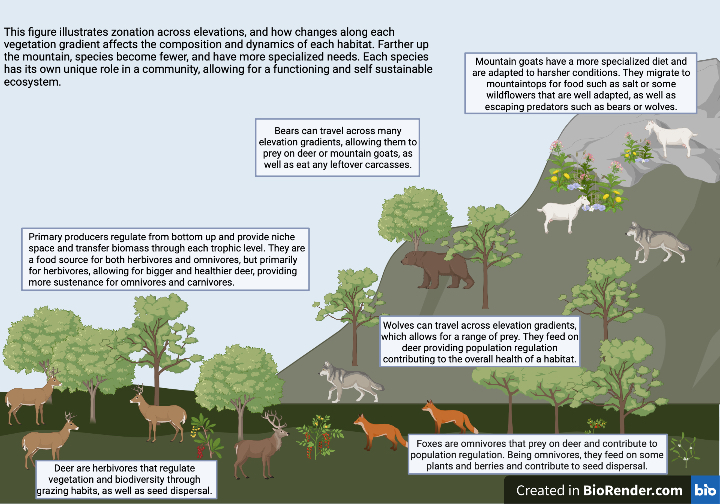
- Association: a type of community with:
- Relatively consistent species composition
- Uniform general appearance
- Distribution that is characteristic of a particular habitat
- Organismic vs continuous concept of communities:
- Organismic:
- There are various discrete organizations of organisms that form communities. Communities are made up of species that are specialized for those specific environmental factors.
- Some generalist species may transcend multiple different discrete communities
- Each cluster of species represents an association and has a similar distribution and abundance peak
- There are various discrete organizations of organisms that form communities. Communities are made up of species that are specialized for those specific environmental factors.
- Organismic:
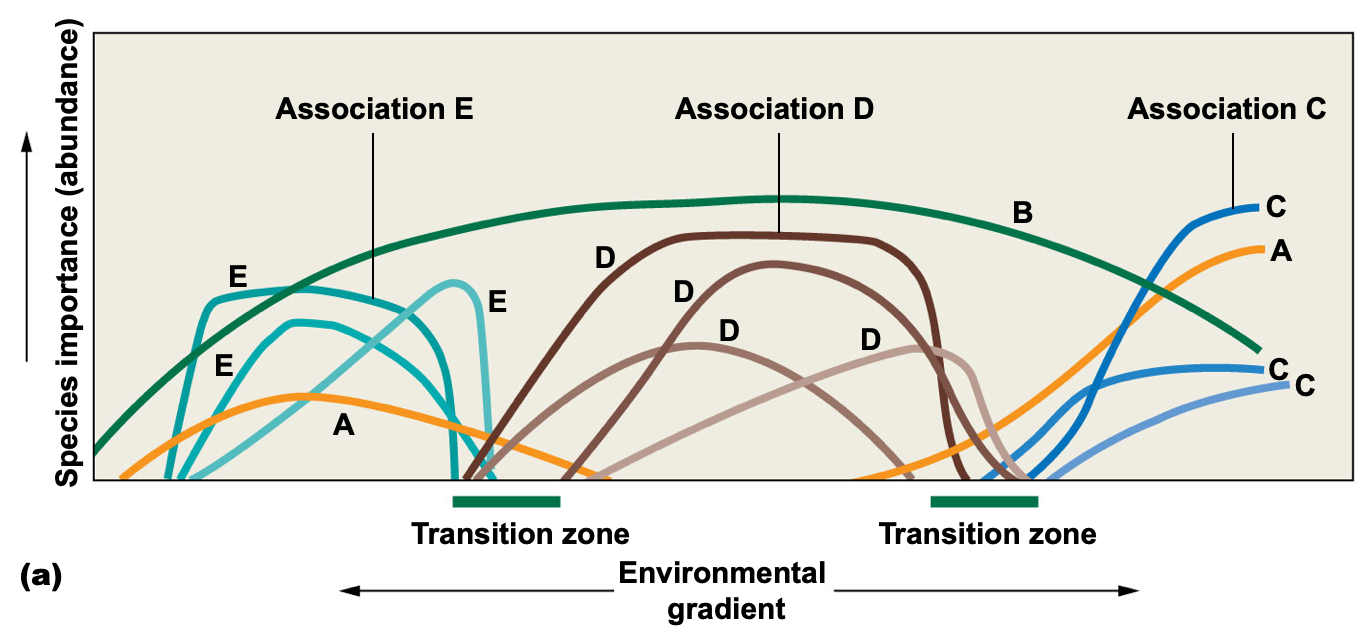
-
- Continuous:
- Community structure changes slowly along an environmental gradient.
- Clusters of species do not exist
- Continuous:
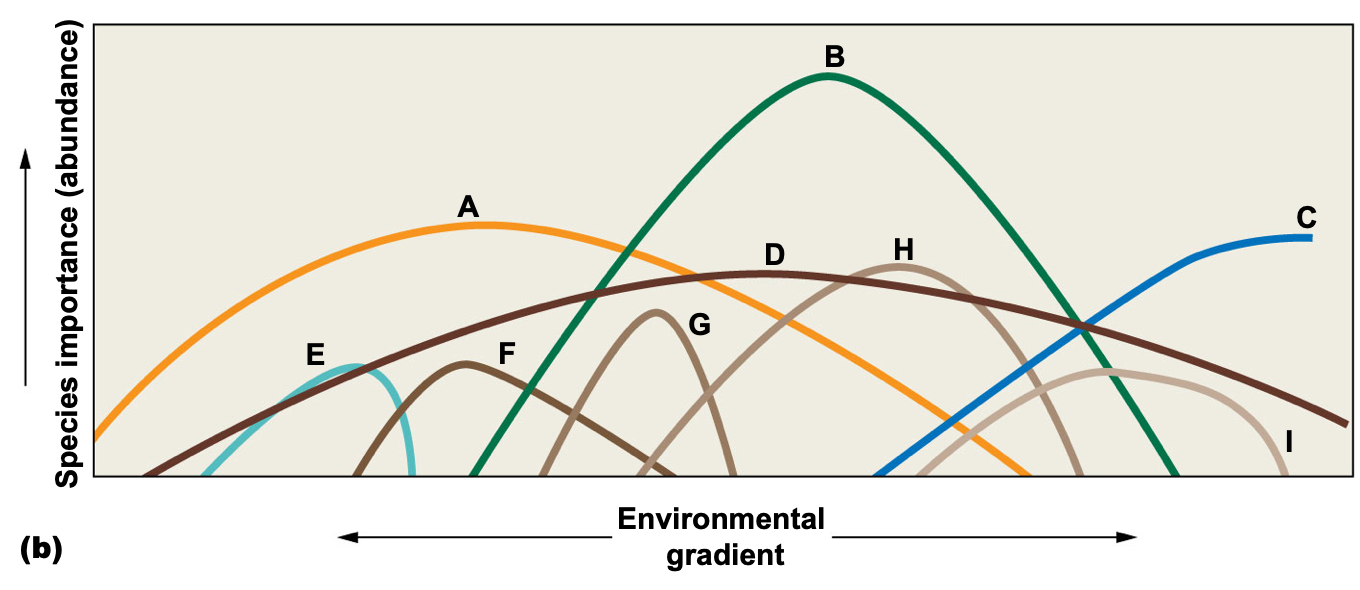
- Succession: patterns in the formation of communities.
- Primary: community has never been established before that develops through pioneer species.
- Ex: concrete or rock environment with lichen
- Pioneer species: highly competitive and opportunistic species; may make the habitat more hospitable (i.e. nitrogen fixation, changing pH levels). Are typically generalist species.
- Secondary: occurs when there is a disturbance, such as a tree fall or hurricane. Differs from primary because it has already been established with species interactions (fungal hyphae, etc.).
- May have the same species composition as the previously established community, but lead to potentially different climax community
- Climax community: the community that forms after a long period of time without major disturbances. Typically comprised of specialist species.
- Primary: community has never been established before that develops through pioneer species.
- Succession of plants also change the animal species composition due to the change in available niches

Key Takeaways
Overarching Themes and Unifying Concepts:
- Communities and changes in community composition are context dependent.
- Changes can be due to temperature, temporal patterns, elevation, or other environmental factors
- Communities change along gradients
- Communities can be made up of associations, with relatively consistent species populations, uniform general appearance, and a distribution that is characteristic of a specific habitat.
- Succession does not just apply to plant communities, but also animal and microbial communities.
- Plant succession allows animal species composition to change as new niches become available.
- These concepts are not always clearly distinguished and commonly have overlap or transitional states.
- Organismic vs continuous communities
How might this information be applied to address grand challenges?
- Anthropogenic interactions can reset or influence all of these ecological processes, specifically in the form of high relative disturbances that do not allow communities to reach their climax state
- We can’t restore every community and new communities trends may develop due to changing anthropogenic forces (increased colonizing species)
- Restoration ecology in areas like prairies, through reduction of invasive species, increase in disturbances, and reducing polluting nutrients
Unsolved Mysteries and Future Research:
- It is currently being studied whether a community that is undergoing secondary succession could lead to a different climax community than the one previously established before the disturbance
- One possible study is the effect of climate change and the increased occurrences of hurricanes as disturbances on the trends of ecological succession within NC forests
- Comparing the western NC to eastern in reference to hurricane paths and effects
- Long-term effects of maintenance disturbances, such as prescribed burns, on community composition
Blog-style Summary:
Communities are an important aspect of ecology, affecting how species interact with each other and the structure of environments. A community is a group of species that interact together, all living in a specific area. These interactions can be either direct or indirect through resource usage, competition, etc. Some communities in nature are wildly varied, with the rank abundance for species depending on the amount of each species present. These species are arranged from most abundant to least abundant, hence “rank” abundance. Communities can also have a species composition that is relatively evenly distributed, uniform, and distributed in a way that is related to their habitats. These kinds of communities are called associations. Vertical structures in communities can be seen from top to bottom, for example how trees are arranged. The canopy layer is the highest layer, the floor is the lowest layer, and the sub-canopy layer is in between. Zonation is the configuration of layers of communities, based on various factors such as changes in elevation or distance from water. The zonation of areas is important because it can help us to understand how communities interact and how the various zones come together.
An example that demonstrates key concepts from this unit is the formation of the Hawaiian rainforest. Hawaii was formed when lava from a hotspot underneath the ocean cooled. Slowly, organisms such as lichens and bacteria were able to grow on the rock, which broke it up and created new soil. These species are called pioneer species because they are hardy enough to survive in a bare environment. Over time, more species are able to grow in the new soil and create simple ecosystems. These ecosystems are called intermediate communities, and they are mostly comprised of generalists. Over time, more species colonize the landscape and form more complex ecosystems with more specialists. These communities are called climax communities because they are relatively permanent. This whole process is called succession, or the process by which stable communities form. The example of the Hawaiian rainforest demonstrates primary succession because the ecosystem formed from previously uncolonized volcanic rock. Secondary succession occurs when a previously colonized community gets wiped out by a disturbance, such as a wildfire or deforestation. Sometimes ecologists create a disturbance to aid species that thrive under such conditions.
Another relevant example is the community structure of rocky intertidal habitats on the West Coast of North America. These communities are characterized by invertebrates that cling to the rocks. The top predator in many of these communities is Pisaster ochraceus, a type of sea star. Pisaster is considered a keystone species, meaning that its presence has a disproportionate effect on the structure of the community. Pisaster mostly feeds on small invertebrates that consume algae. Without Pisaster, these species grow in population, consuming all the algae in an area and taking up physical space. This competition forces some species to leave the system, decreasing the overall richness, or number of species in a community. It also decreases evenness, which describes how evenly distributed species are. Without Pisaster, the relative abundance of a few species increases dramatically, meaning that they consume most of the resources. Often, ecologists will focus on keystone species as a means of benefitting the whole ecosystem.

The importance of understanding the concept of community ecology comes from the various connections between communities. From the start of communities to the present, many processes must occur to reach the point that these communities are at now. As previously mentioned, disturbance is important for some communities to create space for other species. Without disturbance, some communities end up suffering as no new niche space is opened for different species to take hold. Understanding how communities interact allows for proper and effective management to happen. Protecting certain species, such as keystone species, allows communities to thrive because we understand how these species affect the community as a whole. A community is a series of checks and balances that, with improper balancing, could be greatly affected. Management of communities differs between areas and habitats, meaning individual communities need to be studied in order to effectively benefit them.
Restoration ecology relies on knowledge of the community and those ecosystem services, habitat, biodiversity, and economic commodities that sustain life. When we understand the nature and scale of communities and try to learn about their changes over time, like disturbance, our knowledge can increase the chances of restoration success. Increased technology, understanding, interest and resources help address major challenges that stand in the way. These include the scale and magnitude and seemingly nonstop human degradation. Degradation is the process that leads to a condition that is less productive relative to a reference or desirable condition. Restoration is challenging but is an option that can reduce degradation by re-establishing community ecology.
Some examples of restoration ecology in the field are:
- Reclamation of surface mines
- Urban brownfield restoration
- Urban development
Restoration is difficult if not impossible. It is typically very expensive and time-consuming. Even after the implementation of land management decisions, the ecosystem might not ever become or reflect the ecosystem that was previously present. The impacts of mountaintop removal, urban development, and waste management might not be completely erased but it is still important to try. Benayas et al. analyzed 89 restoration sites around the world and found ecological restoration efforts increased biodiversity by 44% and ecosystem services by 25%. Even though these are lower than the intact reference ecosystems, indicators of biodiversity and ecosystem services were positively associated with each other. It is important to remember community ecology and interactions exist on a scale from fungi to top predators. Restoration ecology can help push ecosystems in the right direction allowing the ecosystem to gradually increase its biodiversity. Our environment is constantly changing and as scientists, the more informed on a community scale we are, the better management decisions that can be made for our diverse communities that are constantly pushed to serve more species in smaller landscapes.
Question from a Student:
“I understand that restoration ecology is important and has ties with community ecology. However, restoration ecology is relevant to all areas of ecology, so it is a little confusing why it is talked about more in this section compared to others.”
Answer: Great point. The central goal of restoration ecology is to restore a specific community in an area of interest / concern. So while, as you say, restoration ecology is broadly relevant to a lot of the other topics we cover, it is rooted in community ecology. In fact, we cannot successfully restore and maintain a desired community, if we don’t understand the driving factors and processes that shape the community to begin with.
Spotlight on NC:
History of the Longleaf Pine
A thousand years ago, the area that is now North Carolina was covered in swaths of longleaf pine forests. Over 90 million acres in the southeast supported longleaf pine forest, making them a prominent and critical ecosystem in the region [1]. Longleaf pine forests are an association named after the primary overstory species found in them, longleaf pine (Pinus palustris). The way that longleaf pine grows and the conditions it requires to do so support a huge diversity of other plant and animal species. This diversity is present at both the small and large scale: at a large scale, different regions of the coastal plain harbor community subtypes based on elevation and moisture, resulting in zonation of longleaf pine community types that collectively support hundreds of species–while at the small scale, one square meter of ground in a longleaf pine savanna can harbor 40 species growing in the understory alone [2, 3]. Many of these ground-dwelling species are endemic to the area and specific to this community type, including the venus flytrap (Dionaea muscipula) [3, 4].
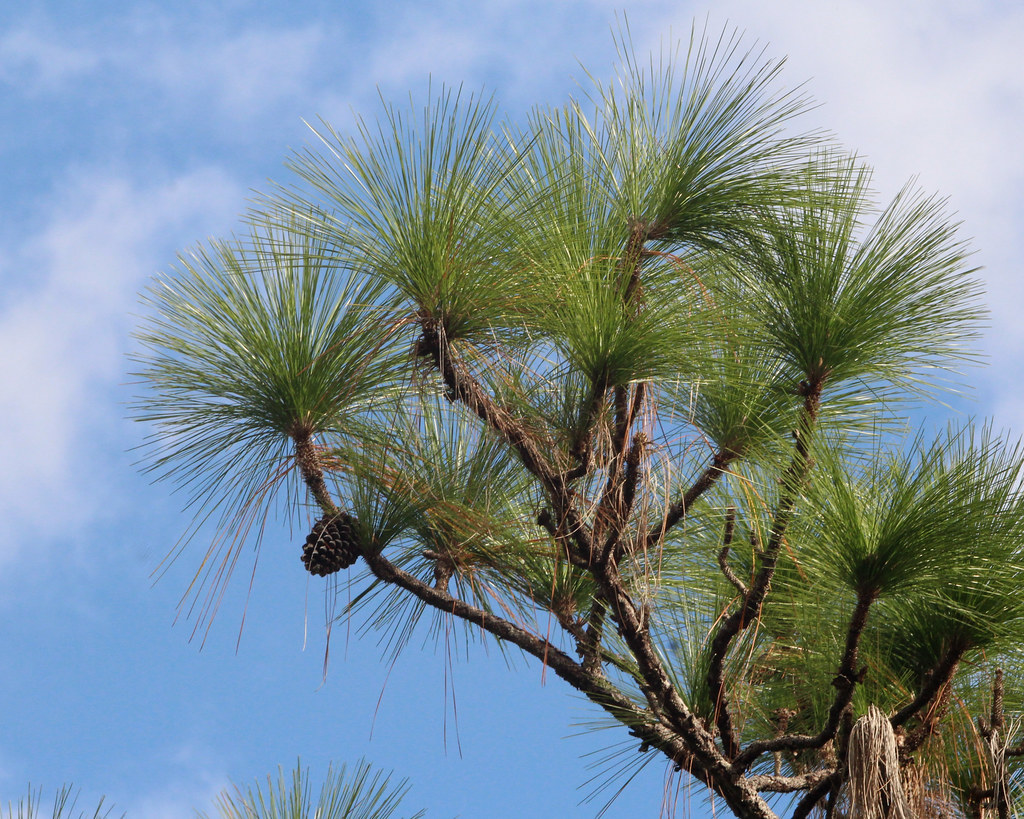
Longleaf pine communities are reliant on disturbance, specifically disturbance caused by fire. Without regular fire, longleaf pines are unable to persist due to competition by other tree species [2]. In this way, longleaf pine communities rely on the suppression of intermediate and climax successional species in order to persist. In these forests the processes and early stages of succession (the turnover of species within a community) are visible: young pines in their pre- and post-fire growth forms populate the understory, surrounded by dense herbaceous and grassy undergrowth and shaded by an open canopy of pines. Burns at the base of the mature trees and charred snags speak to the presence of recent fire that has allowed these young species to establish. This is a constant process, and in this way, the existence of longleaf communities is tenuous and not guaranteed. In fact, longleaf pine forests now total less than 3 million acres.
Venus Flytrap Ecology
The Venus flytrap is a carnivorous plant native to North Carolina coastal plains and sandhills [4]. Though the loamy or sandy soils that can be found in these areas lack nitrogen and phosphorus, venus flytraps make use of their unique snap traps to obtain these nutrients through insect prey and thrive throughout these nutrient-poor ecosystems. These traps are kept close to the ground and simply wait until a crawling insect brushes against at least two of the six trigger hairs of the trap in quick succession (Figure 10) [5]. These species are distinct from pollinator species, which are speculated to be differentiated due to the length of the flowering stem that the flytrap sprouts when it is ready to reproduce (Figure 11) [6].
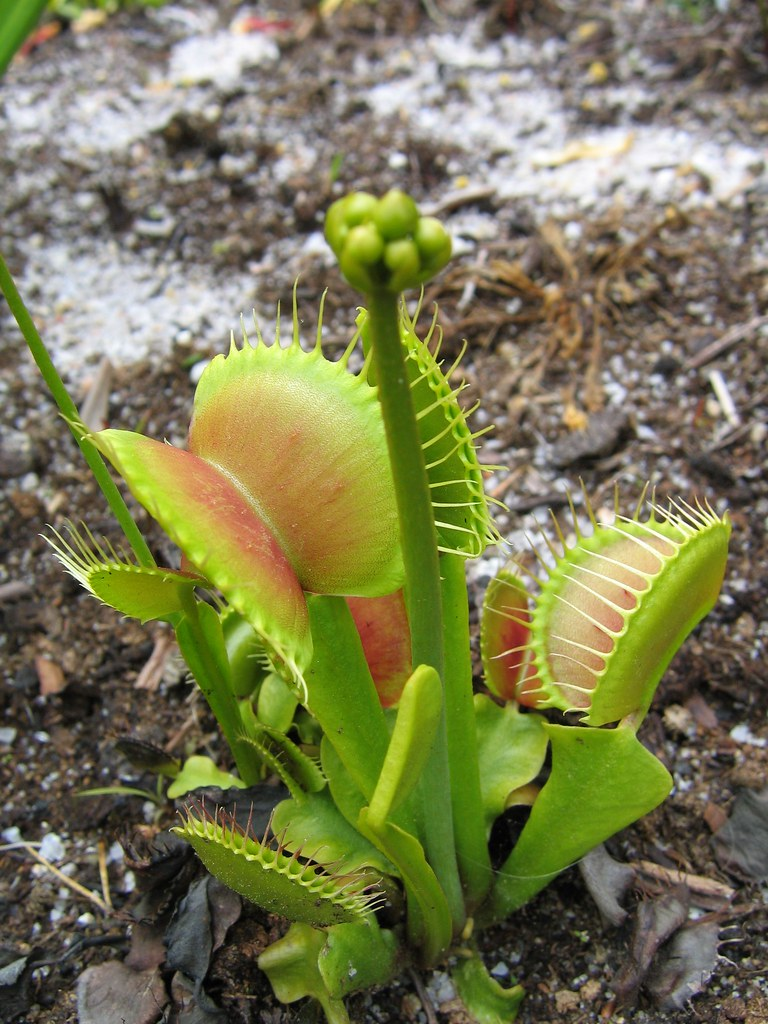
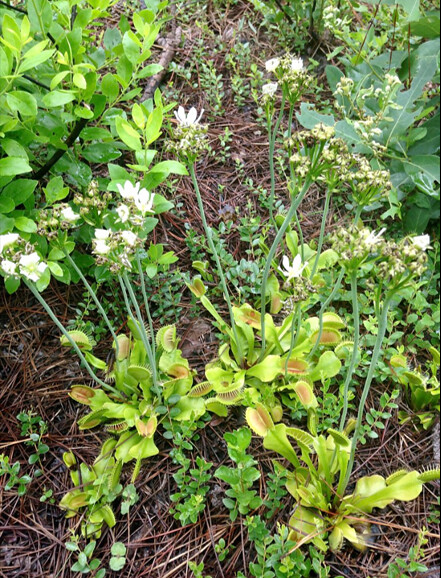
Competition for nutrients is lacking in the soils that the Venus flytrap prefers, so the source of competition they face is from hardier plants overgrowing them. Flytraps are a fire-tolerant species, and rely on regular fire disturbances to burn out the competition and already established flytraps to create more room for seeds to grow [7]. The leftover ash and low canopy cover are the ideal conditions for the seeds to sprout. This makes them a unique pioneer species in successional periods, as many of the first species to appear after a large disturbance like a wildfire would also act to prepare the environment for future colonization. Venus flytraps take little nutrients from the soil, which they are only capable of through their specialized diet.
Threats
Unfortunately, these charismatic plants face a number of challenges that threaten their abundance. In fact, the International Union for Conservation of Nature (IUCN) ranks the Venus flytrap as “Threatened” in their conservation report.
One of the most prominent threats to the species is fire suppression in North Carolina forests (Figure) [4]. This seems odd, right? Wouldn’t allowing forests to burn also destroy the Venus flytrap? Well, not exactly. Since Venus flytraps require an open understory, allowing fires to burn through their forests will remove some of the taller-growing vegetation. Removing this taller-growing vegetation will allow more low-growing plants, like the Venus flytrap, the space to access sunlight and more opportunities to grow. Additionally, by preventing these taller-growing plants from overtaking the forest, the community will have greater richness and evenness. The more that ground-hugging plants are allowed to grow in these forests, the greater the total number of species are able to thrive. The more species there are, the closer the relative abundance (which is a scientific way of saying the proportion of the community that a species takes up) of these species will be.
Additionally, given their unique and compelling nature, Venus flytraps populations are also largely affected by over-collection and poaching, so much so that poaching the plant became a felony in North Carolina in 2014 [4]. By combining this with correctly managing longleaf pine ecosystems, we can continue to protect this special species.
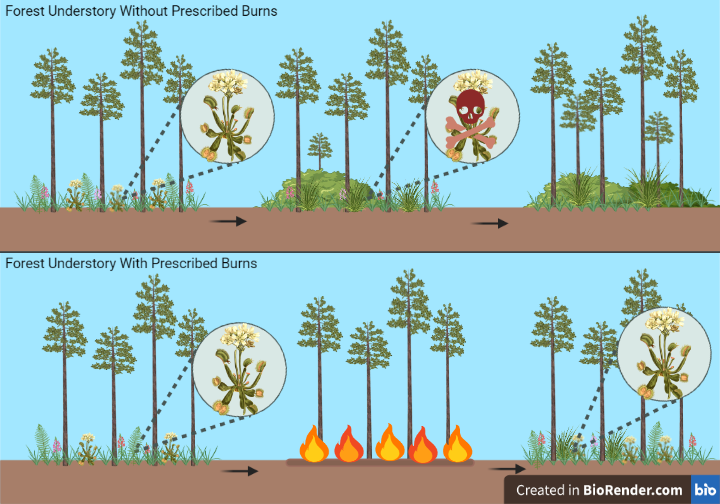
How to Apply Ecology in a Changing World:
Since being introduced to Guam in the 1950s, the Brown Tree Snake (Boiga irregularis) has proved to have harmful effects on local ecological communities [1]. In order to understand these consequences along with management solutions, it is imperative that one first understands the nature of the Brown Tree Snake along with its introduction. The snake is native to Indonesia, the Solomon Islands, New Guinea, and Australia. As a result, they are considered to be a non-native species in Guam. Wherever they are found, they spend most of their time in the trees, making birds and other tree-dwelling creatures susceptible to their predation [2]. In reference to Guam, it is believed that the Brown Tree Snake was introduced shortly after WW2 in cargo ships. Since being introduced, it has caused significant ecological disturbances along with negative socioeconomic impacts. These consequences have resulted from the snake having no natural predators in Guam along with their prey not being appropriately adapted. This has allowed for rapid unchecked reproduction. The University of Washington estimates that every square mile contains 3,000 Brown Tree Snakes resulting in a high relative abundance and species richness on the island [3]. An interesting adaptation of the Brown Tree Snake since being introduced is its ability to “Lasso Climb” [2]. This is when the snake wraps around an electric pole and scales up it with just its body strength; making fewer places for the snakes’ prey to live. Due to its adaptive resilience, rapid reproduction, and negative ecosystem and socioeconomic effects, the Brown Tree Snake has been deemed an invasive species in Guam.
How does the Brown Tree Snake relate to the field of ecology, and why should we care? Well, these kinds of mishaps have happened all throughout history, and there are many parallels to be drawn. Invasive species pose a danger to ecosystems around the world. Without any natural predators, they can outcompete native species for food, space, and other resources. The organisms that fall prey to this invader have no natural mechanisms to defend themselves. This type of competition is consumption, because they outcompete native species for food, disrupting the food web (as shown below in Figure 1). They feed primarily on rats, shrews, and lizards. Shockingly, nine of the eleven most well-known bird species indigenous to the island have gone extinct due to the Brown Tree Snake.

In fact, they have taken such a toll on their environment that they now get most of their food from non-native species, which includes mostly lizards [5]. Brown Tree Snakes have also threatened human health and way of life. With their long length and small diameter, they can slither through cracks and air ducts in houses–inviting themselves as unwelcome guests. There have been over 200 confirmed instances of people being hospitalized for bites [5]. The disproportionate influence that Brown Tree Snakes have on Guam ecosystems has negative consequences that extend into the realm of economics and politics, which are topics we will cover more in-depth in a moment.
But what is the science behind ecological systems plagued by invasive species and how do we remove them? As we’ve found out, invasive species outcompete native species, leading to their decline and possible extinction. This reduction of species richness, or the total number of species in a community, has a terrible impact on the health of the community. Removing invasive species is tricky, since they have few limiting factors. Luckily, there is one thing very few species are ever prepared for: human-mediated environmental change, AKA anthropogenic effects! Scientists can elicit a disturbance to target the occupied habitat to alter or remove a niche. Luckily, there are a few options to do this:
- Biological controls, or introducing a living thing to address the invasive species (example: a mite that only eats a certain type of invasive grass)
- Chemicals, such as pesticides, herbicides, or fungicides
- Manual removal, or physically pulling invasive species from the environment. [6]
All of these options are incredibly risky, incredibly difficult, or an incredible mix of both. For example, there is no way to guarantee a biological control will not just become a new invasive species after the first issue is dealt with without any research. This is partially why the island of Guam has resorted to manual removal of snakes. After the control or removal of invasive species, we can now reintroduce keystone species back into the community. While all of these are ways to “trouble-shoot” invasive species with an ecological perspective, the best solution is to never let an invasive species establish itself in a community in which it doesn’t belong. Unfortunately for the island of Guam, the brown tree snakes are well past that point. So… what now?
Guam is a central trading point and is home to many Air Force bases shipping supplies in and out. Since 1994, traps have been set on airfield fences. Detection dogs have also been trained to prevent snakes from going into military cargo holds. In 2004, the USDA enacted the Brown Tree Snake Eradication and Control Act [7]. The Brown Tree Snake Program drops bait (mice carcasses) that snakes will feed on as opportunistic feeders. The bait contains Tylenol to disrupt the snake’s oxygen carrying ability, ultimately killing them. These drops occur every ninety days and there have been 46,200 drops in five years [6].
References
[1] Colvin, B. A., Fall, M. W., Fitzgerald, L. A., & Loope, L. L. (2005). REVIEW OF BROWN TREESNAKE PROBLEMS AND CONTROL PROGRAMS. USDA National Research Center – Staff Publications. https://digitalcommons.unl.edu/cgi/viewcontent.cgi?article=1627&context=icwdm_usdanwrc
[2] Brown tree snakes in Guam: An overview of the problem and strategies for elimination. (n.d.). Conservation Made Simple. https://www.conservationmadesimple.org/blog/brown-tree-snakes-in-guam-an-overview-of-the-problem-and-strategies-for-elimination
[3] Stricherz, V. (2008, August 21). Brown tree snake could mean Guam will lose more than its birds. UW News. https://www.washington.edu/news/2008/08/21/brown-tree-snake-could-mean-guam-will-lose-more-than-its-birds-2/
[4] Rogers, H. S., Buhle, E. R., HilleRisLambers, J., Fricke, E. C., Miller, R. H., & Tewksbury, J. J. (2017). Effects of an invasive predator cascade to plants via mutualism disruption. Nature Communications, 8(1). https://doi.org/10.1038/ncomms14557
[5] U.S. Department of State. (2009, January 20). Case Study: Brown Tree Snake. U.S. Department of State. https://2001-2009.state.gov/g/oes/ocns/inv/cs/2309.htm.
[6] Control Mechanisms. (n.d.). Invasivespeciesinfo.gov. Retrieved November 14, 2023, from https://www.invasivespeciesinfo.gov/subject/control-mechanisms
[7] Landers, R. (2023, May). Protection and Preservation of Natural Resources Continue with the Eradication of Invasive Brown Tree Snakes. DVIDS. https://www.dvidshub.net/news/444591/protection-and-preservation-natural-resources-continue-with-eradication-invasive-brown-tree-snakes#:~:text=The%20bait%20drop%20is%20an,with%20an%20automated%20bait%20dispenser
[8] Public Law 108 – 384 – Brown Tree Snake Control and Eradication Act of 2004. (2004). https://www.govinfo.gov/app/details/PLAW-108publ384
Featured Ecologists:
E. Lucy Braun, Phd
Dr. Emma Lucy Braun was a prominent botanist and ecologist at the university of Cincinnati. She is best known for writing the 1950 book Deciduous Forests of Eastern North America which is still used by ecologists today. The book was a comprehensive review of the plant species found across the forests of Kentucky, Tennessee, Ohio, Virginia, West Virginia, and Pennsylvania. One topic she was expressly interested in is comparing how these forests used to be, and how human activities have affected them over time, which is something that has come up time and time again in our course. She was the first female president of the Ecological Society of America, and her work to conserve a small limestone prairie in Ohio, called Lynx Prairie, led in part to the establishment of the Nature Conservancy, the largest environmental nonprofit in America. She was an avid plant collector and collected about 12,000 unique specimens for her private herbarium over the course of her life.
Sarah K. Hu
Dr. Sarah Hu’s microbial ecology research focuses on protist communities in the ocean and how protists and other microbial eukaryotes make up a crucial part of the complex ecosystems in the ocean. To understand the role and significance of unicellular eukaryotes, Dr. Hu and her team collect sea water, perform lab incubations, and then study the samples using microscopy, and compare with published data. Dr. Hu studies these communities of unicellular eukaryotes in a variety of habitats, from the coast to deep-sea hydrothermal vents, and at varying depths. She has found that communities change as protists change depths seasonally and time of day depending on sunlight availability, showing how community ecology is not inert. She has also studied parasitic and mutualistic relationships between protists, emphasizing how important these symbiotic relationships are for marine health, determined the phylogenetic relationships between marine protists, and researched the diversity in protists, both in their phylogeny and function. Dr. Hu is publicly a part of the LGBTQ+ community.
Nalini Nadkarni
Dr. Nalini Nadkarni, deemed the “Queen of the Tree Canopy,” is a renowned ecologist known for her work in the field of community ecology of tropical and temperate forest canopies. Community ecology is a topic that we have talked about many times in our class, as we explore the interactions of organisms with their environment. Her work has made significant contributions to our understanding of intricate relationships that exist in complex rainforest ecosystems. Dr. Nadkarni’s research has shed light on the diverse community of plants, animals, and microorganisms that inhabit the canopy of a rainforest in Costa Rica, highlighting their crucial roles in nutrient cycling and forest health. She wanted to understand how plants and animals that live in the canopies interacts with species on the ground. She found that epiphytes intercept nutrients from rainfall, and enhance nutrient cycling in the canopies through forming nutrient-rich organic material on branches. Through methods of canopy access techniques and canopy walkways, she has been able to further advance our scientific understanding of canopy ecosystems and their conservation. Her work has had a lasting impact, emphasizing the importance of preserving these critical yet fragile environments for the well-being of our planet. During her research, Dr. Nadkarni co-founded the International Canopy Network to enhance communication between researchers and educators concerned about forest canopy management, as well as built a database for canopy studies.
Patricia A. Werner, PhD
Dr. Patricia A. Werner researches population and community ecology with a special focus on succession systems. She is especially respected for her plant population ecology. She began her studies researching the survival and growth of trees when put under stress from the environment with the use of fire, grazing, and understory. Dr. Werner mainly focuses on how community ecology functions to support a system that has been damaged by some other factor(s). Her research created a new interest in how plants survive in their natural environments when such disturbances are applied. We studied competition as well as species fitness which is what the majority of Dr. Werner’s research focuses on. Her research allows others to continue what she has started and allows us to understand why certain plant species are able to survive after a disturbance and allows us to find ways to conserve those species that are not able to survive.
Student contributors:
Note Outline: Jacob Hill, Corrine Morales
Blog Style Summary: Ben Regester, Hunter Antel, Claire Melvin
Spotlight on NC: Sydney Beck, Margaret Haney, Lucie Ciccone, Hamilton McInnis
How to Apply Ecology in a Changing World: James Harris, Sally Thompson, Jonah Peckham, Caleb McMurray
Featured Ecologists: Robert Whiting, Hannah Werner
a group of populations of different species that occupy the same area, that interact directly or indirectly
species are listed in order or decreasing relative abundance in a community, with the most abundant species listed first
a type of community with relatively consistent species composition, uniform general appearance, and distribution that is characteristic of a particular habitat (i.e. oak-hickory forest); adapted to specific environmental selective pressures
how biodiversity varies along a vertical gradient. For example, vegetation in a forest shifts from grass and herbaceous ground cover on the forest floor, to shrub and herb layers, to trees that make up the understory and finally the canopy. In a forest, these layers are influenced and determined by the vertical gradient of light.
spatial change in community structure; relates to structure and species composition across a defined area or gradient
highly opportunistic (sometimes "weedy") species that establish themselves during primary succession and may make the habitat more hospitable by fixing nitrogen, changing pH levels, or other "ecosystem engineer" functions
transitional stage of succession characterized by higher turnover of generalist and competitive species
a relatively stable community that forms over a long period of time without major disturbances, whose membership includes species that are adapted to the stressors associated with their given environment
predictable patterns in the formation of communities
primary succession (featuring generalist and opportunistic species), intermediate succession (featuring competitive species), and climax communities (featuring species adapted to specific stressors of a particular environment)
an event that disrupts a community and clears or alters some niches (e.g., hurricane, wildfire)
a species that has a disproportionate effect on the structure of the rest of the community, relative to its abundance in that community
a measure of alpha diversity a measure of alpha diversity that quantifies the number of species in a sample or community of interest
a measure of alpha diversity that quantifies the extent to which species in a community have similar relative abundance
the proportion of individuals in a community that belong to a particular species. The relative abundance of all species in a community add up to equal 1.
returning a habitat, environment, or ecosystem to its original condition or membership, prior to a disturbance (often anthropogenic)